How does fossil support the theory of dirt? This seemingly paradoxical question actually unveils a profound connection between the fossil record and our understanding of Earth’s geological history. Fossils, preserved remnants of past life, are not randomly scattered; their distribution, orientation, and the composition of the surrounding sediment – the “dirt” – provide crucial insights into past environments, climates, and the processes that shaped our planet.
Examining the intricate relationship between fossils and their sedimentary context allows us to reconstruct ancient ecosystems, trace evolutionary lineages, and understand the dynamic interplay between life and the Earth’s surface. This exploration delves into the multifaceted ways in which fossils embedded within various soil compositions illuminate the theory of a constantly changing Earth.
The Artikel meticulously details the processes of fossilization, the types of fossils found in different sedimentary environments, and the techniques used to date these remains. By analyzing the fossil record in conjunction with sedimentological data, we can decipher the chronological order of events, identify past environmental changes (like sea-level fluctuations or shifts in climate), and even reconstruct ancient ecosystems with surprising accuracy.
The inherent biases and limitations of the fossil record are also critically examined, highlighting the challenges and complexities involved in interpreting this invaluable archive of Earth’s history. The study of fossils within the context of the surrounding sediment is not merely about locating and dating specimens; it’s about piecing together a comprehensive narrative of our planet’s dynamic past.
Fossil Formation and Sedimentary Rock
Yo, let’s dive into how fossils get preserved in sedimentary rock – it’s way more rad than you think! We’re talking about the ultimate time capsule, preserving evidence of ancient life for millions of years. This whole process is super complex, influenced by everything from the type of sediment to the environment where the organism died.
Sediment Grain Size and Fossil Preservation
The size of sediment grains – whether it’s tiny clay, silty stuff, sandy bits, or chunky gravel – seriously impacts how well a fossil is preserved. Fine-grained sediments like clay and silt offer better protection because they create a more detailed mold around the organism’s remains. Think of it like wrapping something fragile in bubble wrap – the finer the bubbles, the better the protection.
For example, delicate insect wings are more likely to be preserved in fine-grained shale than in coarse-grained sandstone. Conversely, large, robust bones might survive better in coarser sediments, as they’re less susceptible to being crushed.
Fossilization Processes in Different Sediment Types
Different sedimentary rocks have different stories to tell about fossilization. In claystone, fine-grained clay particles encase the organism, preserving fine details. Sandstone, with its larger grains, might preserve harder parts like bones, but delicate structures are less likely to survive. Shale, similar to claystone, is great for preserving delicate fossils due to its fine grain size. Limestone, often formed from the accumulation of shells and other calcium carbonate, can preserve shells exceptionally well through processes like permineralization, where minerals fill in the pores of the shell.
Diagenesis, the physical and chemical changes that happen after burial, can alter fossils. For example, pressure can flatten shells, and minerals can recrystallize, changing the fossil’s composition.
Fossil Preservation in Various Sedimentary Environments
The environment where an organism dies plays a huge role in its chances of becoming a fossil. Marine environments, like oceans and seas, often have high sedimentation rates and low oxygen levels, which can lead to excellent preservation. Fluvial environments (rivers and streams) have fluctuating oxygen levels and sediment sizes, resulting in variable preservation. Lacustrine (lake) environments can offer good preservation if the lake is deep and oxygen-poor.
Deltaic environments (where rivers meet the sea) are dynamic, with varying sediment types and oxygen levels, leading to a mix of preservation qualities.
Environment | Oxygen Levels | Sedimentation Rate | Water Chemistry | Preservation Potential |
---|---|---|---|---|
Marine (deep ocean) | Low | High | Variable | Excellent for many types of fossils |
Fluvial (river) | Variable | Variable | Variable | Moderate; depends on sediment type and flow |
Lacustrine (lake) | Variable | Low to Moderate | Variable | Good if anoxic conditions prevail |
Deltaic (river mouth) | Variable | High | Variable | Variable; depends on sediment type and mixing |
Stages of Fossil Formation in Sedimentary Rock
This diagram illustrates the steps involved in fossil formation: (Diagram Description): Imagine a series of panels. Panel 1: A dead organism sinks to the bottom of a body of water. Panel 2: Sediment begins to accumulate, burying the organism. Panel 3: Groundwater rich in dissolved minerals percolates through the sediment, filling the pores of the organism’s remains (permineralization). Panel 4: The original material of the organism is gradually replaced by minerals (replacement). Panel 5: A mold is formed as the organism decays, leaving an impression in the surrounding sediment. Later, this mold is filled with sediment to form a cast. Panel 6: A plant is compressed under pressure, losing most of its substance but leaving a carbon film behind (compression and carbonization). Panel 7: Compaction and cementation of the sediment occur over time, hardening the rock and preserving the fossil within it.
Marine vs. Terrestrial Fossil Records
The marine and terrestrial fossil records offer different perspectives on the history of life. Marine environments generally provide a more complete and diverse fossil record than terrestrial environments. This is because the conditions in many marine settings, such as low oxygen levels and rapid sedimentation rates, are favorable for fossilization. Marine fossils commonly include shells, corals, microscopic plankton, and marine vertebrates.
Fossils, remnants of past life, are crucial evidence for understanding the Earth’s history. They illustrate the gradual accumulation of organic matter, forming layers of soil over millennia—a process central to the theory of dirt formation. Understanding this process requires a robust theoretical framework, which is where a deeper understanding of what is knowledge-based theory becomes essential.
Ultimately, the fossil record itself acts as a vast, layered knowledge base, constantly refining our comprehension of how dirt, the very ground beneath our feet, came to be.
However, the marine record is biased toward organisms with hard parts. Soft-bodied organisms are rarely preserved. Terrestrial environments present a different set of challenges. Erosion, scavenging, and decomposition are more common, making preservation less likely. Terrestrial fossils often include bones, teeth, plant remains, and trace fossils like footprints.
The terrestrial record is heavily biased towards organisms that lived in areas with suitable conditions for fossilization, such as floodplains or lakes. The preservation potential also depends on the type of organism, with hard-shelled creatures having a much greater chance of fossilization than soft-bodied organisms. Both records, despite their biases, offer invaluable insights into past life. The terrestrial record provides insights into the evolution of land plants and animals, while the marine record offers information about the evolution of marine life and the changing chemistry of the oceans.
Understanding these biases is critical for accurately interpreting the fossil record and reconstructing past ecosystems.
Fossil Types and Dirt Composition
Yo, let’s dive into how the type of fossil you find is totally linked to the type of dirt it’s chilling in. Basically, different sediments preserve different things, and understanding that connection is key to piecing together ancient ecosystems. Think of it like this: you wouldn’t find a delicate butterfly fossil in a boulder, right? The sediment’s texture and grain size play a huge role in what gets fossilized and how well it’s preserved.Different sediments offer different preservation potentials.
The size and type of sediment particles influence the likelihood of fossil formation and the level of detail preserved in the fossils. Fine-grained sediments, like clay, are more likely to preserve delicate details, while coarser sediments, like sand, might only preserve more robust fossils. Let’s break it down.
Fossil Types and Sediment Grain Size
The relationship between fossil type and sediment grain size is all about preservation potential. Fine-grained sediments like clay and silt have tiny particles that create a more protective environment, ideal for preserving delicate fossils like leaves, insects, and even the fine bones of small animals. Coarser sediments like sand have larger particles, which can damage delicate structures during burial.
As a result, fossils found in sandy sediments tend to be more robust and resistant to damage, like shells or bones of larger animals. Think of it like trying to bury a tiny figurine in sand versus burying it in mud—the mud will protect it better.
Examples of Fossils in Different Sediments
Sediment Type | Grain Size | Fossil Examples | Preservation Quality |
---|---|---|---|
Clay | Very fine | Insects, leaves, fish scales, delicate bone structures | Excellent detail, often showing fine features |
Silt | Fine | Small bones, shells, plant fragments | Good detail, but may lack the finest features of clay fossils |
Sand | Coarse | Shells, large bones, teeth, petrified wood | Good preservation of robust structures, but fine details are often lost |
Dirt Layers and Fossil Stratification

Yo, let’s dive into how layers of dirt, or strata, are like a super awesome timeline of Earth’s history, especially when it comes to fossils. Think of it as a giant, layered cake, each layer representing a different period of time, with the oldest layers at the bottom and the youngest on top. Fossils found within these layers tell us what lived when and how the environment changed over millions of years.
It’s seriously mind-blowing!Geological strata are super important because they show us the chronological order of fossil deposits. Basically, the deeper you dig, the older the stuff gets. This principle, called superposition, is a fundamental concept in geology and helps us understand the sequence of life on Earth. By studying the order of layers and the fossils they contain, we can reconstruct past environments and track the evolution of life.
Fossil Distribution in Strata
Imagine you’re digging up a bunch of fossils. You find trilobites in one layer, then dinosaur bones in a layer above that, and finally, mammal fossils in the top layer. This shows a clear progression of life forms over time. The trilobites, being in the lower layer, are older than the dinosaurs, which are older than the mammals. This isn’t just a guess; it’s backed up by radiometric dating and other methods that confirm the age of the rock layers.
This kind of fossil distribution directly supports the theory of evolution and the vast timescale of Earth’s history.
Environmental Changes Reflected in Dirt Layers
Different dirt layers often indicate different environments. For example, a layer full of sandstone might indicate a desert environment, while a layer packed with shale might represent a lake or swamp. If you find marine fossils in a layer that’s now high above sea level, it suggests that the land has risen or the sea level has dropped significantly over time.
These changes in sediment type and the fossils within them paint a vivid picture of how environments have shifted and changed over millions of years. For example, the presence of coal seams indicates ancient swampy forests, while the presence of limestone suggests a shallow marine environment.
Fossil Stratification and a Changing Earth
The stratification of fossils is solid evidence that Earth is constantly changing. It demonstrates that life forms have evolved over vast periods, with different species appearing and disappearing over time. The changing layers of sediment also show shifts in climate, sea level, and other environmental factors. For instance, finding a layer of glacial till (sediment deposited by glaciers) on top of a layer containing tropical plant fossils demonstrates a dramatic shift from a warm, tropical climate to an ice age.
This kind of evidence is a total game-changer in our understanding of the planet’s dynamic history. It’s not just a static rock; it’s a dynamic record of a planet in constant flux.
Fossil Evidence of Past Environments
Yo, let’s dive into how fossils totally spill the tea on ancient climates and ecosystems. It’s like reading Earth’s super old diary, except instead of angsty teenage entries, we get clues about prehistoric life and environments. We’re talking about how the stuff buried in the ground tells us about the world way back when.
Sedimentary Rock Layers and Fossil Preservation
Sedimentary rocks are the ultimate fossil keepers. Think of them as Earth’s ancient archives. These rocks form from layers of sediment—like sand, silt, and mud—that build up over millions of years. As these layers compact and cement together, they can trap and preserve fossils within them, creating a record of life through time. The different layers, or strata, represent different time periods, making it possible to piece together the story of past environments.
The Principle of Superposition and Relative Fossil Ages
The principle of superposition is a major key. It’s basically saying that in undisturbed rock layers, the oldest layers are at the bottom and the youngest are at the top. This is super helpful for figuring out the relative ages of fossils. If a fossil is found in a lower layer than another, it’s older. It’s like stacking pancakes—the bottom one went in first! This helps us sequence the changes in life and environments over time.
Minerals and Environmental Clues
The minerals in the rock surrounding a fossil can also give us major hints about the ancient environment. For instance, high levels of certain salts in the rock might indicate a salty, ancient ocean or lake. The presence or absence of specific minerals can also reveal clues about oxygen levels in the ancient atmosphere or water. It’s like a detective story, but instead of fingerprints, we’re looking at minerals.
Fossil Assemblages and Ecosystem Reconstruction
Finding multiple fossils together, called a fossil assemblage, is like hitting the jackpot! It allows us to reconstruct entire ancient ecosystems. For example, finding fossils of many different types of marine organisms together tells us that area was once underwater. The types of plants and animals found together reveal information about the climate, food sources, and interactions within that ancient ecosystem.
Fossil Indicators of Specific Environments
Different fossils are like little environmental badges. Certain fossils only appear in specific environments, giving us a clear picture of the conditions in which they lived.
- Aquatic Environments: Trilobites (extinct marine arthropods), Ammonites (extinct shelled cephalopods), Brachiopods (marine invertebrates with shells). Imagine a trilobite, a tiny, segmented creature scuttling along an ancient seabed, or an ammonite gracefully drifting through the water column. The presence of these fossils strongly suggests a marine environment. These fossils are typically found in sedimentary rocks like limestone and shale, which form in underwater settings.
- Terrestrial Environments: Dinosaur bones, fossilized tree trunks, pollen grains. A massive dinosaur bone tells a tale of a land-based ecosystem. The presence of fossilized tree rings can indicate the type of forest that once existed. Pollen grains can reveal the type of plants present in the environment, hinting at the climate and vegetation. These fossils might be found in sandstone, mudstone, or other terrestrial sedimentary rocks.
- Freshwater Environments: Fossilized fish scales and bones from freshwater species, plant fossils adapted to freshwater conditions, insect fossils typical of freshwater habitats. Finding fossils of freshwater fish tells us that the area was once a lake or river. The presence of specific types of plants and insects further confirms the freshwater environment. These fossils are often found in lake sediments or river deposits.
- Marine Environments: Coral fossils, foraminifera (microscopic marine organisms), marine reptile fossils. Coral reefs are indicative of warm, shallow marine environments. Foraminifera are abundant in marine sediments and are sensitive to changes in water temperature and salinity. Marine reptile fossils, like those of plesiosaurs or ichthyosaurs, further support a marine setting. These fossils would be found in limestone, chalk, or other marine sedimentary rocks.
However, it’s not always that simple. The fossil record is incomplete. Many organisms don’t fossilize well, leading to biases in what we find. This is called taphonomic bias—some organisms are just better at becoming fossils than others.
Fossil Type | Indicative Environment | Geological Features |
---|---|---|
Trilobite | Marine | Shale, Limestone |
Dinosaur Bone | Terrestrial | Sandstone, Mudstone |
Fossilized Fish Scales | Freshwater | Lake Sediments |
Coral | Marine | Limestone |
Fossil Evidence Across Geological Periods
Let’s compare some major geological periods: Paleozoic, Mesozoic, and Cenozoic.The Paleozoic Era (541-252 million years ago) was dominated by marine life. Trilobites, brachiopods, and corals were abundant. The Mesozoic Era (252-66 million years ago), the age of dinosaurs, saw the rise of terrestrial ecosystems and large reptiles. The Cenozoic Era (66 million years ago-present) is marked by the diversification of mammals and flowering plants.
Major environmental shifts, like the extinction events at the end of the Permian and Cretaceous periods, are clearly reflected in the fossil record, showing major changes in dominant life forms and ecosystems.
Timeline of Environmental Change: The Grand Canyon
The Grand Canyon in Arizona is a killer example. Its layers expose a vast stretch of geological time, providing a stunning record of environmental changes. The canyon’s rocks contain fossils spanning from the Paleozoic to the Cenozoic, showing shifts in sea level, climate, and vegetation over millions of years. For example, early Paleozoic layers reveal shallow marine environments, while later layers show evidence of terrestrial ecosystems, reflecting the uplift of the Colorado Plateau and the formation of the canyon itself.
Fossil Dating Techniques and Dirt Analysis
Yo, let’s get real about figuring out how old those fossils are and what the dirt they’re chilling in can tell us. We’ve already covered how fossils form and where they’re found, but now we’re diving into the detective work of dating them. It’s like a super-powered archaeological CSI episode, but with way more dirt.Determining the age of fossils involves a dope mix of techniques, and the dirt itself is a major player.
Think of it like this: the dirt is the fossil’s personal time capsule, holding clues about its age and the environment it once called home.
Radiometric Dating
Radiometric dating is the bomb. It uses radioactive isotopes, which are like super-slow-decaying elements, to clock the age of rocks and the fossils within them. Different isotopes decay at different rates, giving us a range of dating methods. For example, carbon-14 dating is great for organic material (like bones or wood) up to about 50,000 years old, while uranium-lead dating can handle rocks billions of years old.
The basic principle is that we measure the ratio of the parent isotope (the original radioactive element) to the daughter isotope (the element it decays into). This ratio, combined with the known half-life (the time it takes for half of the parent isotope to decay), allows us to calculate the age. For instance, if half of the carbon-14 in a sample has decayed, we know it’s been approximately 5,730 years old (carbon-14’s half-life).
Dirt Composition and Fossil Dating
The dirt, or sediment, surrounding a fossil is far from just dirt. It’s a treasure trove of information. The type of sediment – whether it’s sandstone, shale, or limestone – can tell us about the environment where the fossil formed. For example, fossils found in marine sediments indicate a past ocean environment. Furthermore, the layers of sediment, or strata, provide a relative timeline.
Fossils found in deeper strata are generally older than those in shallower strata. This principle, called superposition, is a fundamental concept in geology. Analyzing the chemical composition of the dirt can also provide clues. For instance, the presence of certain minerals or isotopes can help correlate the fossil-bearing layer with other dated layers in the area, refining the age estimate.
Say you find a fossil in a layer with a specific volcanic ash deposit that’s been dated using radiometric methods. That date then helps constrain the age of your fossil.
A Flowchart for Fossil Dating
Imagine a flowchart. It starts with discovering the fossil. Next, you assess the surrounding sediment layer and its position relative to other layers (superposition). Then, you collect samples of the sediment and the fossil itself. This is followed by radiometric dating of appropriate samples (carbon-14, uranium-lead, etc., depending on the suspected age).
Simultaneously, you analyze the dirt’s composition – identifying minerals, isotopes, and the type of sediment – for additional clues and correlation with other dated layers. Finally, you integrate all the data to obtain the most accurate age estimate for the fossil. This might involve comparing your results with other fossils found in similar strata or using statistical methods to combine data from multiple dating techniques.
Fossil Distribution and Geographical Location
Yo, let’s dive into how fossils are spread across the globe and what that tells us about Earth’s history. It’s not random, fam – the location of fossils gives us major clues about how continents have moved and changed over millions of years. Think of it like a giant, ancient puzzle where the pieces (fossils) reveal the bigger picture (plate tectonics).Fossil distribution patterns reveal a lot about past environments and the movement of continents.
For instance, finding the same types of fossils on different continents that are now far apart suggests those continents were once connected. This evidence is seriously crucial for understanding the theory of plate tectonics and continental drift. The presence of specific fossils in certain rock layers also helps us date those layers and reconstruct past ecosystems.
Continental Drift’s Influence on Fossil Location
Continental drift, the slow movement of Earth’s tectonic plates, is a major player in where we find fossils today. Imagine a supercontinent, Pangaea, existed millions of years ago. As Pangaea broke apart, landmasses drifted, carrying fossils with them. So, similar fossils found on continents separated by oceans today are evidence of their shared past. For example, the discovery of
- Mesosaurus*, a freshwater reptile, in both South America and Africa supports the idea that these continents were once joined. If
- Mesosaurus* couldn’t swim across the Atlantic, its presence on both continents points to a time when they were connected. The distribution of Glossopteris flora, a group of plants, across southern continents like Australia, Antarctica, India, and South America, also provides compelling evidence for continental drift. These plants couldn’t have spread across such vast oceans, but their shared presence suggests they thrived on a single, interconnected landmass.
Fossil Distribution and Plate Tectonics
Fossil distribution provides powerful support for the theory of plate tectonics. The geographic distribution of fossils is inconsistent with the current arrangement of continents. However, when we consider the past movement of tectonic plates, the distribution makes perfect sense. For example, the discovery of similar marine fossils in mountain ranges on different continents provides strong evidence that these mountain ranges were once located underwater and connected.
The alignment of geological formations and fossil distributions across continents strongly suggests the past existence of a single supercontinent, Pangaea, and its subsequent breakup and movement. The fit of the continents, like puzzle pieces, combined with the fossil evidence, forms a compelling case for plate tectonics.
Map Illustrating Fossil Distribution
Imagine a world map. Let’s focus on a few examples. In the southern continents (South America, Africa, Australia, Antarctica, and India), we’d see widespread markings indicating the distribution of
- Lystrosaurus*, a Triassic therapsid (a reptile-like mammal ancestor). This highlights the connection of these continents during the Triassic period. Across the northern continents (North America, Europe, and Asia), we might highlight the distribution of
- Tyrannosaurus rex* fossils, showing its prevalence in a specific region during the Late Cretaceous. The map would visually represent the geographic distribution of different fossils across continents, highlighting patterns that support the theories of continental drift and plate tectonics. The map would clearly demonstrate how the current distribution of fossils can only be explained by considering the past movements of continents.
It wouldn’t be a simple dot map; instead, it would incorporate shaded regions to illustrate the broader distribution of fossil types across continents. This visual representation would strongly support the connection between fossil distribution, continental drift, and plate tectonics.
Microscopic Fossils in Dirt
Yo, digging into dirt isn’t just about getting your hands dirty – it’s about unlocking secrets from the past! Microscopic fossils, or microfossils, are tiny but mighty time capsules that reveal tons about ancient environments and the organisms that thrived (or didn’t) in them. These little guys are way more common than their bigger, macrofossil cousins, and they provide a seriously detailed picture of Earth’s history.Microfossils offer invaluable insights into past climates and ecosystems.
Their presence, abundance, and types tell us about everything from ancient ocean temperatures and salinity to the types of plants and animals that lived millions of years ago. Think of them as the ultimate historical detectives, leaving behind clues that help us reconstruct entire ancient worlds. By analyzing their morphology (shape and structure) and chemistry, we can piece together a pretty comprehensive story of past life and the conditions it experienced.
Microfossil Types and Their Environmental Significance
Different types of microfossils thrive in specific environments. For instance, diatoms, single-celled algae with intricate silica shells, are abundant in aquatic environments. Their presence in a soil sample might indicate a past lake or ocean. Conversely, pollen grains, microscopic reproductive structures of plants, are indicative of terrestrial environments and can be used to reconstruct past vegetation patterns.
Foraminifera, single-celled organisms with calcium carbonate shells, are found in both marine and freshwater environments and are extremely sensitive to changes in water temperature and salinity. Their shell shapes and isotopic compositions can be used to reconstruct past oceanographic conditions with remarkable accuracy. Spores, released by fungi and other organisms, also offer clues about past environments, reflecting the types of plants and other organisms present.
The diversity and abundance of these different microfossil types provide a detailed picture of the past ecosystem.
Comparing Macrofossils and Microfossils
Macrofossils, like dinosaur bones or fossilized leaves, are pretty rad, but they tell only part of the story. They provide information about large organisms, but they’re relatively rare. Microfossils, on the other hand, are way more abundant and diverse, providing a much more complete picture of past ecosystems. While macrofossils might show us what a giant sloth looked like, microfossils can reveal the entire ecosystem the sloth lived in – from the types of plants it ate to the microorganisms in the soil.
Think of it like this: macrofossils are like finding a single piece of a puzzle, while microfossils are like finding thousands of pieces, giving you a much clearer image of the whole picture.
Examples of Microfossils in Different Dirt Types
Let’s get specific. In a sample of soil from a former swamp, you might find abundant pollen from water-loving plants, along with diatoms and other aquatic microorganisms. A soil sample from a dry, desert environment might contain abundant pollen from drought-resistant plants, alongside other drought-tolerant microorganisms. Soil from a marine environment will likely be rich in foraminifera, diatoms, and other marine microfossils.
The types and abundance of microfossils found in a given soil sample help scientists accurately reconstruct the environmental conditions that existed when that soil was formed. These tiny fossils, often overlooked, are actually key players in understanding Earth’s deep time.
Fossil Preservation and Taphonomy: How Does Fossil Support The Theory Of Dirt
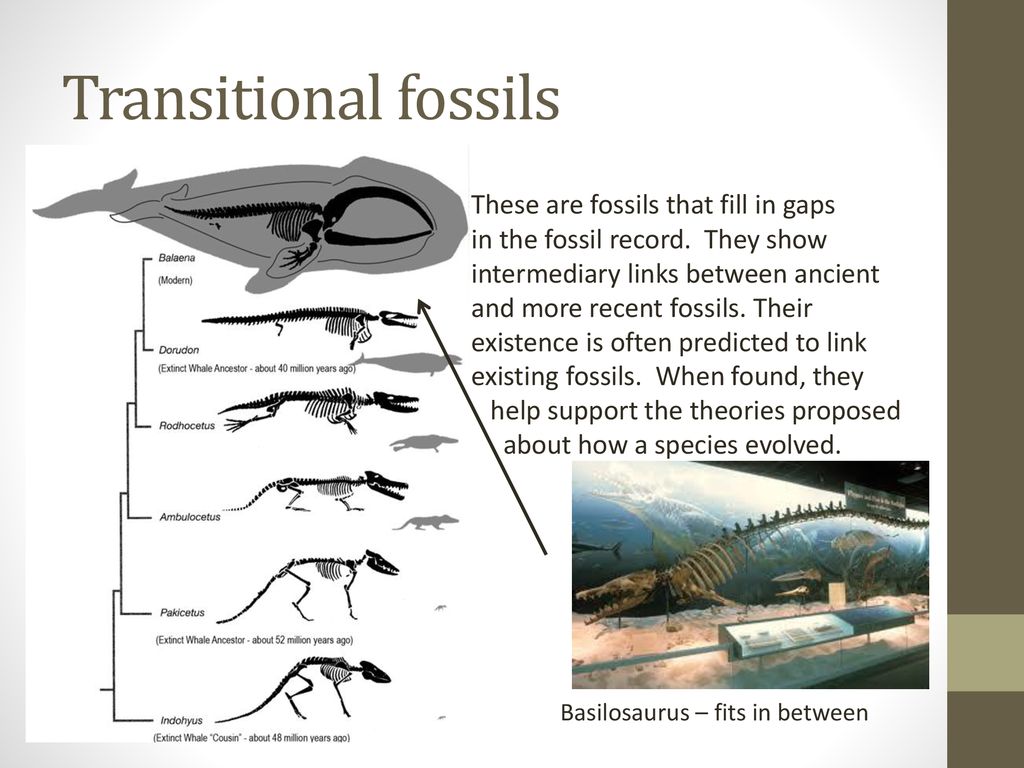
Yo, let’s dive into how fossils get preserved – it’s way more complex than you think! Fossil preservation isn’t just about a dino getting buried; it’s a whole chain of events, from death to discovery, that determines what survives and what doesn’t. We’re talking taphonomy, the study of what happens to an organism after it dies and before it becomes a fossil.
It’s like the ultimate post-mortem investigation for ancient life.Fossil preservation is a total crapshoot, seriously. Many factors determine whether a creature becomes a fossil or just decomposes. Think of it like a race against time – decay versus preservation. The speed and type of burial, the environment’s chemistry, and even the organism’s own composition all play huge roles in whether it’ll become a fossil that future paleontologists can geek out over.
Factors Influencing Fossil Completeness
Several factors determine how complete a fossil is. Rapid burial is key – quick burial protects the remains from scavengers and the elements, increasing the chances of preservation. The organism’s hard parts, like bones and shells, are way more likely to fossilize than soft tissues, which usually decay quickly. The sediment type also matters; fine-grained sediments like mud or clay are better at preserving delicate details than coarse sediments like sand.
Think of it like molding clay versus trying to make a detailed sculpture out of gravel. Environmental conditions, such as oxygen levels and the presence of certain chemicals, also affect preservation. Low oxygen environments, like swamps or bogs, can slow down decay and increase the chances of preserving soft tissues.
Taphonomy’s Role in Fossil Interpretation
Taphonomy isn’t just about how fossils form; it’s about interpreting what we find. Paleontologists use taphonomic evidence to understand the processes that affected the fossils after death. For example, if a fossil shows signs of scavenging or transport, that tells us something about the environment and the events that followed the organism’s death. It’s like reading a detective novel where the clues are scattered across millions of years.
Analyzing these clues helps us to reconstruct past ecosystems and understand the biases in the fossil record. It’s a total puzzle, and taphonomy gives us the tools to put the pieces together.
Burial Environments and Fossil Preservation
Different burial environments have vastly different effects on fossil preservation. Marine environments, like oceans and seas, often lead to excellent preservation due to the fine-grained sediments and low-oxygen conditions. Many marine fossils are beautifully preserved, showcasing intricate details. Terrestrial environments, on the other hand, can be more challenging. Fossils found in terrestrial settings are often more fragmented due to scavenging and erosion.
Volcanic eruptions can create unique preservation environments, sometimes preserving organisms in ashfalls that act like giant, natural molds. Think Pompeii, but with dinosaurs instead of people. Each environment has its own unique set of challenges and opportunities for fossil preservation, adding complexity and nuance to the paleontological puzzle.
Fossil Evidence and Evolutionary History
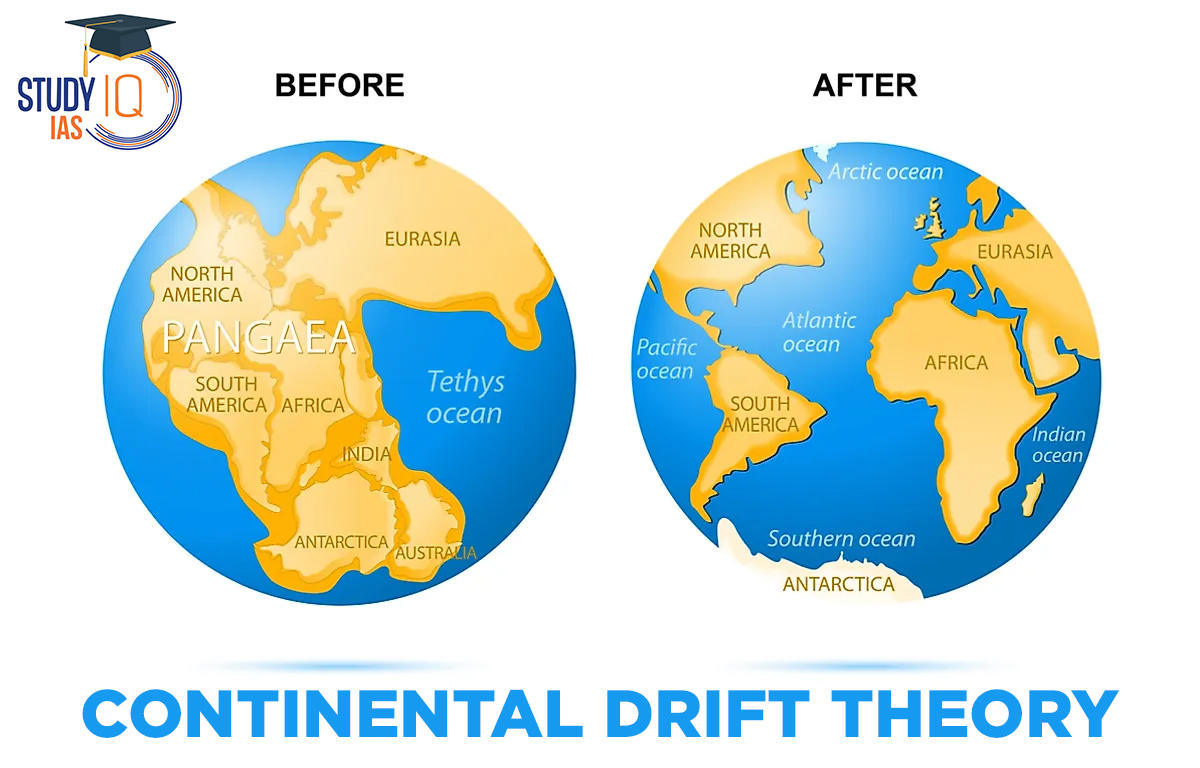
Fossils are like time capsules, preserving clues about life on Earth millions of years ago. By studying them, we can piece together the story of evolution, tracing how life has changed and diversified over time. The arrangement of fossils within different rock layers provides a powerful record of the history of life, revealing the rise and fall of species and the evolution of new traits.
Relative Ages of Organisms and Evolutionary Lineages
The principle of superposition, a cornerstone of geology, states that in undisturbed rock layers, the oldest layers are at the bottom and the youngest are at the top. This means that fossils found in lower layers are generally older than those in higher layers. This provides a relative dating system, showing the sequence of life’s appearance, but not the exact age in years.
Index fossils, which are fossils of organisms that existed for a relatively short period and were geographically widespread, are particularly useful for correlating rock layers across different locations. By identifying the same index fossil in different rock formations, paleontologists can determine that those layers are of the same age. For instance, the presence of a specific trilobite species might indicate a particular period in the Paleozoic Era.
Examples of Transitional Fossils
Transitional fossils document the intermediate stages between ancestral and descendant groups, providing strong evidence for evolutionary transitions.
- Archaeopteryx: This iconic fossil, dating back to the Late Jurassic period (approximately 150 million years ago) and discovered in Germany, represents a transitional form between dinosaurs and birds. Key features include reptilian teeth and a long bony tail (reptilian characteristics), alongside feathers and a wishbone (avian characteristics).
- Tiktaalik: Discovered in Canadian Arctic rocks from the Late Devonian period (about 375 million years ago), Tiktaalik exhibits characteristics of both fish and early amphibians. Its robust, weight-bearing fins and primitive wrist bones are intermediate structures between fins and limbs, representing a crucial step in the transition from aquatic to terrestrial life. Its flattened head and neck are also significant departures from typical fish anatomy.
- Ambulocetus: This “walking whale” from the Eocene epoch (about 50 million years ago), discovered in Pakistan, bridges the gap between land mammals and whales. Its hind limbs were adapted for both swimming and walking, and its skull shows features intermediate between those of land mammals and modern whales. The presence of involucrum, a bony structure in the ear region, characteristic of aquatic mammals, further supports its transitional nature.
Fossil Record and Gradual Change
The fossil record often shows gradual changes in morphology within lineages over long periods. For example, the evolution of the horse is well documented by a series of fossils showing a gradual increase in size, lengthening of limbs, and reduction in the number of toes, reflecting adaptation to open grasslands. However, the fossil record is incomplete; many organisms don’t fossilize, and many fossils haven’t been discovered.
This can lead to gaps in our understanding of gradual evolutionary change, potentially creating an illusion of punctuated equilibrium (rapid evolutionary change followed by periods of stasis).
Visual Representation of Evolutionary Changes
[Imagine a cladogram here. The base would be a common ancestor, branching off to show five species: 1. An early tetrapod (e.g.,
- Ichthyostega*), characterized by its limbs and lungs. 2. A more derived tetrapod (e.g.,
- Eryops*), showing improved limb structure. 3. An early reptile (e.g.,
- Hylonomus*), showing scales and amniotic eggs. 4. A therapsid (e.g.,
- Cynognathus*), showing mammalian characteristics like differentiated teeth. 5. An early mammal (e.g.,
- Morganucodon*), showing fully developed mammalian characteristics. The cladogram would show the evolutionary relationships based on shared derived characteristics, such as the presence of limbs, amniotic eggs, and differentiated teeth.]
Comparison of Fossilization Methods
Process | Type of Fossils Produced | Environmental Conditions | Examples of Organisms |
---|---|---|---|
Permineralization | Mineralized remains, often preserving fine details | Burial in sediment with groundwater rich in minerals | Petrified wood, dinosaur bones |
Mummification | Preserved soft tissues and skin | Extremely dry environments or anoxic conditions | Wooly mammoths, insects in amber |
Analysis of a Hypothetical Fossil Discovery
If a new fossil were discovered, paleontologists would follow a series of steps: 1) Initial description and documentation, including location and geological context. 2) Dating using radiometric methods (e.g., carbon-14, potassium-argon) to determine the fossil’s age. 3) Comparative anatomy studies to identify similar features in other organisms, living or extinct. 4) Phylogenetic analysis using cladistics to determine the fossil’s evolutionary relationships.
This new fossil could potentially fill a gap in the fossil record, providing crucial information about a particular evolutionary lineage, such as the origin of a key adaptation or the timing of a speciation event.
Comparison of Fossil Evidence for Evolutionary Transitions
The fossil record for the evolution of flight in birds shows a gradual transition from feathered dinosaurs with increasingly adapted wings to the modern avian form. Key fossils like
Archaeopteryx* document intermediate stages in this process.
In contrast, the fossil record for the evolution of whales from land mammals demonstrates a more dramatic transformation. Fossils like
- Pakicetus* and
- Ambulocetus* show a progressive adaptation to an aquatic lifestyle, with a gradual reduction of hind limbs and changes in skeletal structure. The transition was relatively rapid compared to the evolution of flight in birds.
Essay: The Significance of the Fossil Record in Understanding Evolutionary History
The fossil record, though incomplete, stands as a monumental testament to the history of life on Earth, providing irrefutable evidence for evolution. Its significance lies in its ability to document the sequence of life’s appearance, reveal transitional forms linking ancestral and descendant groups, and illuminate the gradual changes in morphology over time. However, understanding the fossil record requires acknowledging its inherent limitations.
Taphonomy, the study of fossilization, highlights the biases involved in fossil preservation. Certain organisms and environments are more likely to be fossilized than others, leading to an incomplete picture of past biodiversity. Furthermore, many fossils remain undiscovered, creating gaps in the evolutionary narrative.Despite these limitations, the fossil record provides compelling support for evolutionary theory. The discovery of
- Archaeopteryx*, a transitional form between dinosaurs and birds, showcases the gradual evolution of flight. Similarly, the fossil record documents the transition from land mammals to whales, with fossils like
- Pakicetus* and
- Ambulocetus* illustrating intermediate stages. The evolution of horses, as documented by a series of fossils showing changes in limb structure and tooth morphology, demonstrates adaptive radiation in response to environmental changes. The Cambrian explosion, revealed through the sudden appearance of diverse body plans in the fossil record, illustrates a period of rapid diversification. Finally, the discovery of
- Tiktaalik*, a transitional form between fish and amphibians, illuminates the critical step in the colonization of land.
While the fossil record is far from complete, its strengths lie in its ability to provide concrete evidence of past life and evolutionary relationships. By combining fossil evidence with molecular data and other lines of evidence, we can construct a more comprehensive understanding of evolutionary history. The ongoing discovery and analysis of fossils continue to refine and enrich our understanding of the intricate tapestry of life’s evolution on Earth.
Dirt Composition and Fossil Degradation

Fossil preservation is a complex dance between the remains of ancient life and the ever-changing chemistry of the surrounding soil. The composition of dirt, its physical and chemical properties, and the processes of weathering and erosion all play crucial roles in determining whether a fossil will survive the passage of time or crumble into dust. This section delves into the intricate relationship between dirt composition and the degradation of fossils.
Mineral Composition and Fossil Degradation
Different minerals in soil exhibit varying chemical properties that significantly impact fossil degradation. Quartz, for instance, is chemically inert and relatively resistant to alteration, offering little direct effect on fossils. In contrast, clay minerals, often possessing high surface areas and cation exchange capacities, can chemically interact with fossil materials, leading to alteration or dissolution. Carbonates, like calcite, can undergo dissolution in acidic conditions, affecting shell fossils.
Mineral Type | Chemical Properties | Impact on Bone | Impact on Shell (Calcite) | Impact on Plant Fossils |
---|---|---|---|---|
Quartz (SiO2) | Inert, insoluble | Minimal effect | Minimal effect | Minimal effect |
Clay Minerals (e.g., Kaolinite) | High surface area, cation exchange | Potential for chemical alteration | Potential for dissolution in specific conditions | Can accelerate decomposition |
Calcite (CaCO3) | Soluble in acidic conditions | Minimal direct effect | Dissolution in acidic soils | Can be incorporated into the fossil, or dissolve |
Organic Matter Content and Fossil Degradation
The abundance and type of organic matter in soil significantly influence fossil degradation rates. Humus, a complex mixture of decomposed organic materials, provides a rich environment for microbial activity. These microbes, through their metabolic processes, can break down both organic matter and fossil components. High organic matter content often correlates with faster decomposition rates due to increased microbial activity.
Conversely, soils low in organic matter may lead to slower degradation, depending on other factors.
Soil pH and Redox Potential and Fossil Preservation
Soil pH and redox potential are crucial factors governing the solubility and stability of fossil materials. Acidic soils (low pH) tend to accelerate the dissolution of carbonate-based fossils like shells and can also affect bone preservation through the leaching of minerals. Conversely, alkaline soils (high pH) can enhance the preservation of some materials. Redox potential (Eh), reflecting the availability of oxygen, influences the oxidation state of minerals.
For example, iron-rich minerals in bone can be oxidized in oxygen-rich environments, leading to their alteration and potentially impacting preservation.
Physical Weathering of Fossils
Physical weathering processes, such as freeze-thaw cycles and abrasion, can mechanically break down fossils. Freeze-thaw, where water expands upon freezing, creates stress within fossils, leading to fracturing. Abrasion, caused by the movement of sediment or water, can wear down fossil surfaces, especially in smaller or more delicate fossils. Larger, more robust fossils are less susceptible to these effects.
Chemical Weathering of Fossils
Chemical weathering involves chemical reactions that alter fossil composition. Dissolution, where minerals dissolve in water, affects soluble components like carbonates. Oxidation involves the reaction of minerals with oxygen, leading to changes in their chemical state, as seen in the rusting of iron-containing minerals in bone. Hydrolysis, where water molecules react with minerals, can break down silicate minerals.
Dissolution of Calcite: CaCO3(s) + H+(aq) → Ca2+(aq) + HCO3-(aq)
Oxidation of Iron: 4Fe2+(aq) + O2(g) + 4H+(aq) → 4Fe3+(aq) + 2H2O(l)
Erosion and Fossil Degradation
Erosion processes, including water, wind, and ice, transport sediment and can further degrade fossils. Water erosion can physically abrade fossils and dissolve soluble components. Wind erosion can expose fossils to further weathering and transport them to new locations. Glacial erosion can cause significant physical damage and transport fossils over large distances. The rate of erosion is influenced by soil properties such as cohesiveness and permeability, as well as the surrounding landscape.
Impact of Acidic Soils on Fossil Preservation
Acidic soils accelerate fossil degradation primarily through the dissolution of minerals. The increased concentration of H+ ions in acidic soils promotes the dissolution of carbonates and can leach minerals from bone, leading to structural weakening and eventual disintegration.
Acid Rain and Fossil Degradation
Acid rain, caused by atmospheric pollutants, significantly impacts soil chemistry. The increased acidity of rainwater lowers soil pH, enhancing the dissolution of fossils and altering the soil environment in ways that accelerate degradation.
Mitigation Strategies for Acidic Soil Effects
Mitigation strategies for acidic soil effects on fossil preservation include buffering agents to neutralize soil acidity and controlled environments to minimize exposure to damaging elements. These methods aim to slow or prevent the deterioration of fossils in acidic conditions.
Case Study 1: Dinosaur Bone Preservation
Dinosaur bones found in alkaline soils often exhibit better preservation than those in acidic soils. The alkaline environment inhibits the dissolution of bone minerals, leading to better preservation of bone structure and internal details. In contrast, acidic soils lead to significant mineral loss and structural damage.
Case Study 2: Shell vs. Bone Preservation
Fossil Type | Composition | Preservation in Neutral Soil | Preservation in Acidic Soil |
---|---|---|---|
Shell | Calcite (CaCO3) | Good | Poor (dissolution) |
Bone | Calcium phosphate | Good | Moderate (mineral leaching) |
This table highlights that even within the same soil environment, different fossil types exhibit varying degrees of preservation due to their inherent chemical compositions.
Geographic Variation in Soil Chemistry and Fossil Preservation
The map would visually represent how differences in soil chemistry across various geographic locations significantly influence fossil preservation rates and patterns. Areas with consistently acidic soils, for instance, might show significantly lower fossil preservation rates than areas with consistently alkaline or neutral soils. This variation highlights the crucial role of geology and regional environmental factors in shaping the fossil record.
Fossil Chemistry and Dirt Analysis
Yo, let’s dive into the seriously cool world of fossil chemistry and how it spills the tea on past environments and creatures. By analyzing the chemical makeup of fossils and the dirt they’re buried in, we can unlock secrets about ancient climates, diets, and migration patterns – it’s like detective work, but way more prehistoric.Chemical Analysis of Fossils and Past EnvironmentsThis section breaks down how the chemical fingerprints of fossils reveal clues about where and how they lived.
We’re talking trace elements, element ratios—the whole shebang.
Chemical Markers in Fossilized Bone and Environmental Interpretation
Analyzing the chemical composition of fossilized bone can tell us a lot about the environment the animal lived in. Different elements and their ratios act as unique identifiers. For instance, higher concentrations of strontium often indicate a marine environment, while certain trace elements might point to freshwater sources.
Element | Concentration Range (Example) | Environmental Interpretation |
---|---|---|
Strontium (Sr) | High | Marine environment |
Fluorine (F) | High | Groundwater influence |
Oxygen (O) isotopic ratios | Vary depending on water source | Indicates freshwater vs. marine or other water sources. |
Chemical Composition of Fossilized Plant Material and Soil Properties
The chemical makeup of fossilized plant material, like leaf cuticles, can reveal details about the soil it grew in. For example, the presence of certain elements can indicate soil pH, nutrient levels, and even the frequency of past fires. High levels of certain elements might suggest nutrient-rich soil, while others could indicate acidic or alkaline conditions. Charred remains, of course, are a direct indicator of fire.
Chemical Differences Between Fossilized Herbivore and Carnivore Teeth
Herbivore and carnivore teeth have distinct chemical signatures. Carnivore teeth often show higher concentrations of elements like zinc and phosphorus, reflecting their diet rich in bone and muscle tissue. Herbivore teeth might have different ratios of these elements and higher concentrations of elements reflecting their plant-based diet. The differences stem from the different types of tissues they consume and how those tissues are processed.Isotopic Analysis of Fossils and Dirt AnalysisIsotope analysis is like a super-powered magnifying glass for fossils.
By looking at the ratios of different isotopes within fossils and the surrounding dirt, we can gain insights into ancient climates, migration patterns, and even dietary habits.
Carbon-13 Analysis of Fossilized Bone Collagen and Soil Organic Matter
Comparing the Carbon-13 (δ¹³C) values in fossilized bone collagen and surrounding soil organic matter can reveal dietary habits and ecosystem dynamics. Discrepancies might arise due to factors like dietary diversity or the influence of other carbon sources in the soil. Analyzing both provides a more complete picture.
Oxygen Isotope Ratios in Fossilized Teeth and Past Climate Reconstruction
A flowchart visualizing the process:Sample Collection –> Fossil Preparation –> Isotope Extraction –> Mass Spectrometry Analysis –> δ¹⁸O Calculation –> Paleoclimate Interpretation (Temperature, Precipitation).Limitations include potential alteration of isotopic ratios during fossilization and the need for robust calibration against independent climate proxies.
Strontium Isotope Ratios in Fossils and Animal Migration
The strontium isotope ratio (⁸⁷Sr/⁸⁶Sr) in fossils can reveal migration patterns. Animals absorb strontium from their environment, and different geological regions have distinct strontium isotope signatures. By comparing the strontium isotopes in fossils to those in the surrounding geology, scientists can track the movements of ancient animals. Challenges include potential isotopic mixing and the need for detailed geological maps.Stable Isotopes and Past Climate ConditionsStable isotopes are like time capsules, preserving information about past climates.
By analyzing their ratios in fossils and sediments, we can reconstruct ancient environmental conditions.
δ¹⁸O Values in Foraminifera and Global Ice Volume
The δ¹⁸O values in fossilized foraminifera (single-celled marine organisms) are closely linked to global ice volume. During glacial periods, more water is locked up in ice sheets, leaving the remaining ocean water enriched in the heavier ¹⁸O isotope. This relationship is used to reconstruct past sea levels and climate change events.
Multiple Stable Isotope Systems and Paleoenvironmental Reconstruction (Amazon Basin Pleistocene Example)
Multiple stable isotope systems (δ¹³C, δ¹⁵N, δ¹⁸O) provide a holistic view of past environments. In the Amazon basin during the Pleistocene, analyses revealed shifts in vegetation, water availability, and animal diets, reflecting climate changes and ecosystem dynamics.
Chemical Analysis of Fossils and Dietary HabitsChemical analysis can also reveal what ancient creatures ate!
Nitrogen Isotope Ratios in Fossilized Bone Collagen and Trophic Level Determination
The δ¹⁵N values in fossilized bone collagen reflect the animal’s trophic level (position in the food chain). Higher δ¹⁵N values generally indicate higher trophic levels, meaning the animal was a predator or higher up the food chain. Limitations include potential variations due to diet composition and the possibility of isotopic fractionation during the fossilization process.
Trace Element Analysis in Fossilized Teeth and Dietary Habits of Extinct Hominins
Diet | Trace Element Signature (Examples) |
---|---|
Herbivore | High Sr, low Zn |
Carnivore | High Zn, low Sr |
Omnivore | Intermediate levels of Sr and Zn |
Stable Carbon Isotope Ratios in Fossilized Plant Tissues and Herbivore Dinosaur Diets
The δ¹³C values in fossilized plant tissues can distinguish between C3 and C4 plants. C3 plants (most trees and shrubs) have lower δ¹³C values than C4 plants (grasses). By analyzing the δ¹³C values in the bones of herbivorous dinosaurs, we can infer the types of plants they consumed.
Paleontological Techniques and Dirt Sampling
Yo, digging up dino bones and ancient pollen ain’t just about grabbing a shovel and digging. It’s a serious science requiring precise techniques to get accurate results. From microscopic fossils to massive bones, the methods used are super specific, and getting it wrong can totally mess up your findings. Let’s break down the process.
Fossil Collection and Analysis Techniques
Collecting fossils, whether they’re tiny pollen grains or giant dinosaur femurs, requires different approaches. For macrofossils like bones and shells, careful excavation with brushes, trowels, and picks is key. We’re talking precision, yo! The goal is to remove the surrounding sediment without damaging the fossil. For microfossils like pollen and diatoms, however, things get a little more intricate.
We use sieving, a process of separating materials based on size, to isolate the fossils from the surrounding sediment. Flotation techniques, which use liquids of different densities to separate fossils from the sediment matrix, are also crucial. Chemical preparation methods, involving the use of acids to dissolve away surrounding rock, are sometimes employed, but this needs to be done carefully to avoid damaging the fossils.
Sampling Methods in Paleontological Studies
Proper sampling is crucial for minimizing contamination and getting a representative sample of the fossil record. Imagine trying to understand a whole population based on only talking to one person! Stratified random sampling divides the area into strata (layers) and randomly selects samples from each. This ensures a diverse representation. Grid sampling, on the other hand, involves establishing a grid over the area and systematically collecting samples at each grid point.
This is super helpful for mapping fossil distributions. Both methods have their pros and cons depending on the geological context. For example, stratified sampling is best for areas with visible layering, while grid sampling is better for more homogenous areas. Recording GPS coordinates, stratigraphic position (where the fossil is located within the rock layers), and associated sediment characteristics for each sample is essential for accurate data analysis and interpretation.
This provides crucial context.
Fossil Preparation and Preservation
Preparing and preserving fossils is an art and a science. Fragile fossils, like delicate plant fossils, require gentle cleaning and often need consolidants to strengthen them. Consolidants are like glue that strengthens the fossil without altering its structure. Robust fossils, like large bones, might require more aggressive cleaning techniques. Adhesives are used to repair damaged fossils, while casting materials can create replicas for study and display.
Proper storage conditions, including controlled temperature, humidity, and minimal light exposure, are essential for long-term preservation. Think of it like keeping your favorite sneakers in pristine condition – you wouldn’t leave them out in the sun and rain! Ethical considerations are paramount; fossils are part of our shared heritage, so responsible collection and preservation practices are non-negotiable.
Step-by-Step Guide for a Paleontological Dig
Let’s break down a typical paleontological dig into phases:
- Pre-Dig Planning: Secure the necessary permits (legalities are crucial, dude!), research the site thoroughly (know your history!), develop a detailed sampling strategy, and gather all the necessary equipment and personnel (teamwork makes the dream work!).
- Excavation: Establish a grid system for precise location tracking, carefully excavate sediments layer by layer (don’t rush!), document the stratigraphy meticulously (note the layers!), photograph and record all findings (pics or it didn’t happen!).
- Sample Processing: Sieve, wash, and conduct initial fossil identification in the field (get a head start!).
- Laboratory Analysis: Detailed cleaning, consolidation, preparation, and precise identification of fossils (time for the deep dive!).
- Data Analysis and Reporting: Statistical analysis of fossil data, interpretation of results, and the preparation of a final report (sharing is caring!).
Sediment Types and Fossil Preservation
Different sediment types offer varying levels of fossil preservation potential.
Sediment Type | Grain Size | Porosity | Permeability | Fossil Preservation Potential |
---|---|---|---|---|
Clay | Fine | Low | Low | Good for delicate fossils, but can hinder access |
Silt | Medium | Moderate | Moderate | Moderate preservation |
Sand | Coarse | High | High | Good for hard parts, but can damage delicate fossils |
Gravel | Very Coarse | High | High | Generally poor preservation, unless fossils are robust |
Selecting Appropriate Sampling Techniques
(A flowchart would be visually represented here, showing decision points based on fossil type and geological context. The flowchart would branch out based on factors like the size of the fossils, the type of sediment, and the research questions. For example, if microfossils are sought, it would lead to techniques like sieving and flotation. If large fossils are expected, it would direct to careful excavation techniques.)
Ethical paleontological practice demands respect for cultural heritage, adherence to all relevant laws and regulations, and responsible data sharing to ensure the preservation of our planet’s history for future generations. Unauthorized collection is a major issue, and responsible reporting is key to protecting the fossil record for future scientific inquiry.
Impact of Climate Change on Paleontological Fieldwork
Climate change poses significant challenges to paleontological fieldwork. Increased frequency of extreme weather events like floods and droughts can damage fossil sites, making excavation more difficult and dangerous. Melting permafrost in Arctic regions exposes new fossils but also threatens their preservation due to increased erosion and degradation. Changes in sea levels can also impact coastal fossil sites.
Interpreting Fossil Evidence in Context
Yo, digging up dino bones is way cooler than it sounds, but interpreting what you find? That’s where the real brainpower comes in. It’s not just about finding fossils; it’s about understanding the whole story behind them – the challenges, the biases, and the techniques used to piece together the past. Let’s break it down.
Challenges in Interpreting Fossil Evidence Found in Dirt
Fossil interpretation isn’t a walk in the park. Diagenesis, the chemical and physical changes that happen to sediment after deposition, can seriously mess with fossils. Imagine a perfectly preserved T-Rex skull slowly dissolving due to groundwater acidity – bummer! Taphonomy, the study of what happens to an organism after death, adds another layer of complexity. Bones can be scattered, crushed, or even eaten by scavengers before they fossilize, leaving incomplete or distorted remains.
Shells might dissolve completely depending on the pH of the surrounding environment, while plant remains can get compressed and carbonized, losing intricate details. Distinguishing between fossils that were buried where the organism died (in situ) and those moved after death (reworked) is crucial for understanding past environments. A cluster of marine fossils found high in the mountains is a clear sign of tectonic uplift, a story completely different from the same fossils found on a modern beach.
Incomplete preservation is another huge challenge. Finding a single, isolated bone gives you a limited view compared to a fully articulated skeleton, drastically impacting our ability to reconstruct the organism’s appearance, size, and behavior. Think of trying to understand a whole car by only finding one tire.
Importance of Considering Geological Context When Analyzing Fossils
Stratigraphy, the study of rock layers, is key to dating fossils. Older layers are typically found beneath younger ones (the principle of superposition). Relative dating uses this principle to establish the order of events. Absolute dating techniques, like radiometric dating (using radioactive isotopes), provide numerical ages. Biostratigraphy uses index fossils—fossils with known ages and widespread distribution—to date rock layers.
Sedimentary structures like bedding planes, cross-bedding, and ripple marks are like clues about past environments. Cross-bedding, for example, indicates flowing water, helping us reconstruct ancient rivers or dunes. Tectonic processes—earthquakes, mountain building, and erosion—can move fossils around, making it tricky to understand their original location and distribution. A fossil found on a mountaintop today might have originally lived near the coast millions of years ago.
How Biases Can Influence the Interpretation of Fossil Data, How does fossil support the theory of dirt
Scientists aren’t immune to biases. Confirmation bias—favoring evidence that supports pre-existing beliefs—can lead researchers to overlook contradictory data. Anchoring bias, clinging to initial interpretations even when presented with new evidence, is another common pitfall. For example, an initial interpretation of a fossil as belonging to a particular species might persist even when further analysis reveals differences. Pre-existing theoretical frameworks can guide research but also limit interpretations.
If a researcher firmly believes in a particular evolutionary pathway, they might interpret fossil evidence to fit that model, potentially overlooking alternative explanations. A researcher’s background and beliefs can subtly influence their interpretations. Strategies to mitigate bias include blind analysis (analyzing data without knowing its context), multiple independent analyses, and rigorous peer review.
Examples of How Misinterpretations of Fossil Evidence Can Be Avoided
Minimizing bias and improving accuracy requires a multi-pronged approach. Rigorous methodology is essential—meticulous documentation, precise measurements, and statistical analysis are non-negotiable. Interdisciplinary collaboration—geologists, geochemists, and paleontologists working together—provides a broader perspective and enhances the reliability of interpretations. For instance, a team combining paleontological expertise with geochemical analysis of the surrounding rocks could better understand the depositional environment and the processes that affected fossil preservation.
Technique | Application | Limitations |
---|---|---|
Blind analysis | Analyzing data without prior knowledge of the sample’s context | May not always be feasible |
Multiple independent analyses | Comparing interpretations from different researchers | Can be time-consuming and expensive |
Peer review | Subjecting interpretations to scrutiny by experts | May not always identify all biases |
Fossil Trace Fossils and Dirt
Yo, let’s dive into the seriously cool world of trace fossils – the clues left behind by ancient organisms, not their actual bodies. These aren’t just bones; we’re talking burrows, footprints, poop – the whole shebang. They’re super important because they tell us about how ancient creatures lived, moved, and interacted with their environment. Think of them as the ancient world’s Instagram feed, showing us what life was like back in the day.
Trace Fossil Description and Classification
Trace fossils found in dirt are mostly from invertebrates, like worms, arthropods, and mollusks. These fossils record their activities – think digging, crawling, munching, and even resting. Three prime examples are burrows, which are tunnels dug by organisms; trails, which are markings left by movement across a surface; and borings, which are holes made by organisms in hard substrates.
Trace Fossil Type | Description | Typical Organism Producer | Preservation Method |
---|---|---|---|
Burrow | Tunnel dug into sediment | Worm, crustacean | Sediment infilling and lithification |
Trail | Markings left by movement on a surface | Snail, arthropod | Sediment infilling and lithification |
Boring | Hole drilled into hard substrate (e.g., shell, rock) | Bivalve, sponge | Mineralization or infilling |
Taphonomic processes – how fossils get preserved – are crucial for trace fossils. Several factors influence preservation:
- Sediment type: Fine-grained sediments preserve delicate traces better than coarse ones.
- Depositional rate: Rapid burial preserves traces before they’re destroyed by erosion or bioturbation (mixing by other organisms).
- Diagenetic alteration: Changes in the sediment after burial (e.g., cementation, compaction) can affect preservation, sometimes enhancing or destroying the trace.
Behavioral Insights from Trace Fossils
Trace fossils are like a window into the past behavior of extinct organisms. For example:
- Feeding strategies: The shape and size of feeding structures (like borings) indicate what kind of food the organism consumed.
- Locomotion: Trails and footprints reveal how organisms moved – their gait, speed, and even whether they walked, crawled, or swam.
- Habitat preferences: The location and type of trace fossils can indicate where organisms lived and the conditions they preferred (e.g., burrows in wet environments vs. trails on dry surfaces).
Different trace fossil types reveal distinct behavioral aspects:
Trace Fossil Type | Behavioral Inference | Example Organism (if known) | Limitations in Interpretation |
---|---|---|---|
Burrow | Social behavior, environmental conditions (oxygen levels, water content) | Various invertebrates | Difficult to link definitively to a specific species |
Footprint | Gait, speed, body size estimation | Dinosaurs, other vertebrates | Footprint shape can be ambiguous, erosion can distort the size |
Resting trace | Posture, vulnerability | Trilobites, other invertebrates | Interpretation relies on comparative anatomy and analogy to modern organisms |
Trace Fossils vs. Body Fossils
Trace and body fossils offer different perspectives on the past:
Feature | Trace Fossils | Body Fossils |
---|---|---|
Preservation Potential | Generally higher, less susceptible to decay | Lower, dependent on conditions for preservation |
Information Yielded | Behavior, ecology, environment | Morphology, anatomy, phylogeny |
Bias in Fossil Record | Underrepresented compared to common organisms | Favors organisms with hard parts, abundant populations |
Combining trace and body fossils provides a much more complete picture of past ecosystems than using either type alone. Body fossils tell us
- what* lived, while trace fossils tell us
- how* they lived.
Case Study: The Ediacaran Biota
The Ediacaran biota, found in various locations worldwide, presents a fascinating assemblage of trace fossils from the late Precambrian. These fossils, often preserved as impressions in sandstone, represent a diverse range of organisms with unique locomotion and feeding strategies. The inferred paleoenvironment was a shallow marine setting, with evidence of both mobile and sessile organisms. The traces provide crucial insights into the early evolution of animal life and the development of complex ecosystems.
(Source: Seilacher, A. (1953).
Die fossilen Fußspuren der Wirbellosen.* Gustav Fischer Verlag.)
Future Research Directions in Fossil-Dirt Interactions
Yo, digging deeper into the fossil-dirt connection is where it’s at. We’ve learned a ton about how fossils form and what they tell us about the past, but there’s still a whole universe of unanswered questions waiting to be cracked. Future research needs to focus on refining our methods and tackling some seriously mind-bending mysteries.The relationship between fossils and the dirt they’re buried in is way more complex than we initially thought.
Understanding this interaction is key to unlocking a more complete picture of Earth’s history and the evolution of life. New technologies are opening up exciting avenues for research, allowing us to analyze fossils and their surrounding sediments with unprecedented precision. This allows for more accurate interpretations of the past.
Advanced Imaging Techniques
High-resolution X-ray computed tomography (CT) scanning and other advanced imaging techniques are revolutionizing paleontology. These non-destructive methods allow researchers to create detailed 3D models of fossils embedded in rock, revealing intricate anatomical details that would be impossible to see otherwise. For example, CT scans have allowed scientists to visualize the internal structures of fossilized insects trapped in amber, revealing details of their soft tissues and even their last meals.
This level of detail provides insights into the organism’s life and the environment it inhabited. Furthermore, advanced imaging can reveal subtle changes in the surrounding sediment, providing clues about the conditions under which the fossil was buried and preserved.
Geochemical Analysis of Fossil-Sediment Interactions
The chemical composition of both the fossil and the surrounding sediment holds a wealth of information about the environment at the time of burial. Sophisticated geochemical analyses can reveal details about water chemistry, temperature, and even the presence of specific organisms. For instance, stable isotope analysis of fossil shells can provide insights into the temperature and salinity of ancient oceans.
Similarly, analysis of trace elements in the surrounding sediment can reveal clues about the type of environment in which the organism lived and died. Future research will focus on developing more sensitive and precise geochemical techniques to extract even more information from these materials.
Microbial Interactions and Fossil Preservation
Microorganisms play a crucial role in the decomposition and preservation of fossils. Understanding how microbial communities interact with organic matter during fossilization is essential to interpreting the taphonomic history of a fossil. Future research will likely focus on investigating the specific roles of different microbial groups in fossil preservation, potentially using techniques like metagenomics to analyze the microbial communities associated with fossils.
This could lead to a better understanding of why some fossils are exquisitely preserved while others are highly degraded. For example, researchers are exploring the role of certain bacteria in the formation of authigenic minerals that replace bone, preserving intricate details of the skeletal structure.
Developing Predictive Models of Fossil Preservation
By combining data from various sources, including taphonomic studies, geochemical analysis, and advanced imaging, researchers can begin to develop predictive models of fossil preservation. These models could help paleontologists to better understand the factors that influence the likelihood of fossil discovery and the quality of preservation. This, in turn, could guide future fieldwork and improve the efficiency of fossil discovery.
For instance, a model might predict the likelihood of finding well-preserved vertebrate fossils in certain types of sedimentary rock formations based on factors like sediment grain size, depositional environment, and the presence of specific minerals.
The Application of Machine Learning
Machine learning algorithms are increasingly being used in various scientific fields to analyze large datasets and identify patterns that might be missed by human observation. In paleontology, machine learning could be used to automate the identification and classification of fossils, to analyze large datasets of fossil locations and environmental data, and to predict the likelihood of fossil preservation in different geological settings.
For example, machine learning algorithms could be trained on large datasets of fossil images to automatically identify and classify different species of dinosaurs. This would greatly speed up the process of fossil analysis and allow researchers to analyze much larger datasets.
Top FAQs
What are some common misconceptions about fossil preservation?
Many believe fossilization is a common occurrence, but it’s actually a rare event. Also, the idea that fossils always represent complete organisms is inaccurate; most fossils are incomplete or fragmented.
How do fossils help us understand past climate change?
Fossil assemblages, combined with isotopic analysis of the surrounding sediment, reveal past climates. For example, the presence of certain plant fossils indicates past temperatures and precipitation levels.
What is the significance of index fossils?
Index fossils are widespread, easily identifiable fossils that existed for a relatively short period. They are crucial for correlating rock layers of the same age across different geographic locations.
How does continental drift affect fossil distribution?
Continental drift explains the presence of similar fossils on continents now separated by vast oceans, supporting the theory of plate tectonics and past land connections.