A History of the Theories of Aether unveils a captivating saga of scientific inquiry, spanning millennia. From ancient Greek conceptions of a fundamental substance permeating the cosmos to its pivotal role in 19th-century physics, the aether hypothesis profoundly shaped our understanding of light, space, and time. This exploration delves into the evolution of aether theories, from philosophical musings to scientifically testable hypotheses, culminating in their ultimate demise with the advent of Einstein’s relativity.
We will examine key figures, pivotal experiments, and the enduring legacy of this once-central concept in physics.
The journey begins with the ancient Greeks, whose philosophical notions of a celestial medium laid the groundwork for later scientific interpretations. We then traverse the Scientific Revolution, charting the contributions of Descartes and Newton, and witness the rise of the luminiferous aether in the 19th century, a concept attempting to explain the propagation of light. The Michelson-Morley experiment, a landmark in scientific history, dramatically challenged the aether hypothesis, leading to a paradigm shift in our understanding of the universe.
The narrative continues through the development of Maxwell’s equations and the Lorentz-FitzGerald contraction, highlighting the attempts to reconcile the aether with electromagnetism. Finally, we explore the triumph of Einstein’s theory of relativity, which rendered the aether concept obsolete, and briefly touch upon alternative post-relativity aether theories.
Ancient Greek Conceptions of Aether
The concept of aether, a fundamental substance permeating the universe, held a central place in ancient Greek philosophy, shaping their understanding of the cosmos and influencing scientific thought for centuries. Early thinkers grappled with the nature of reality, seeking to explain the seemingly empty space between celestial bodies and terrestrial matter. Their diverse models, while often lacking the rigor of modern physics, provided a fertile ground for later scientific investigations into the nature of space.The pre-Socratic philosophers, seeking to understand the fundamental building blocks of the universe, offered various interpretations of the aether.
Anaximander, for example, posited an “apeiron,” an undefined and boundless substance from which all things arose. While not explicitly termed “aether,” this concept foreshadowed the idea of a fundamental, all-pervading medium. Other philosophers, like Empedocles, proposed a combination of elements—earth, air, fire, and water—to explain the composition of the universe, but the precise role of a fifth element, akin to aether, remained undefined in their systems.
These early attempts, although rudimentary, laid the groundwork for more sophisticated models of the universe.
Aristotle’s Aether and its Distinctions from Other Models
Aristotle’s influence on the concept of aether was profound and long-lasting. Unlike the pre-Socratic philosophers who focused on the material composition of the universe, Aristotle’s model emphasized the celestial realm as distinct from the terrestrial. He proposed a fifth element, “aether,” which constituted the heavens and possessed properties unlike the four terrestrial elements. Aristotle’s aether was incorruptible, unchanging, and moved in perfect circular motion, unlike the terrestrial elements which were subject to change and decay.
This stark distinction between the terrestrial and celestial realms, based on the properties of aether, shaped cosmological thought for centuries. In contrast to the more fluid and less defined conceptions of aether in pre-Socratic thought, Aristotle’s aether was a precisely defined substance with specific properties and a unique role in the cosmos. This provided a framework for understanding the apparent perfection and regularity of celestial motion.
The Enduring Legacy of Ancient Greek Aether Theories
The ancient Greek conceptions of aether, particularly Aristotle’s model, exerted a considerable influence on subsequent scientific thought. The idea of a fundamental substance filling the universe persisted through the Middle Ages and into the Renaissance, often interwoven with religious and philosophical interpretations. Later scientific investigations into light and other phenomena frequently built upon, or reacted against, the framework established by the ancient Greeks.
For instance, the development of the luminiferous aether in the 19th century, a hypothetical medium for the propagation of light, shows the enduring legacy of the ancient Greek concept. While ultimately proven incorrect, the luminiferous aether theory demonstrates the persistent appeal and influence of the ancient Greek notion of a fundamental, all-pervading substance in the universe. The search for a medium to explain the propagation of light was directly inspired by the earlier concept of aether, highlighting the lasting impact of these ancient ideas on the development of modern physics.
Aether in the Scientific Revolution
The Scientific Revolution witnessed a dramatic shift in the understanding of the universe, and the concept of aether played a pivotal role in this transformation. No longer simply a philosophical notion, aether became a crucial element in scientific models attempting to explain the physical world, particularly the propagation of light and the mechanics of celestial bodies. This period saw the emergence of competing theories, each proposing a different nature and function for this elusive substance.
Descartes and Newton, two giants of 17th-century science, offered contrasting views on the nature of aether. Descartes, a proponent of a mechanistic worldview, envisioned aether as a subtle fluid filling all space, responsible for transmitting forces and facilitating the motion of celestial bodies. This plenum, as he termed it, was a continuous, perfectly elastic medium, vital to his understanding of the universe’s workings.
In contrast, Newton, while acknowledging the potential existence of aether, was less reliant on it in his explanations of gravity. His theory of universal gravitation, while powerful, left the mechanism of gravitational action somewhat unexplained, a gap that some later scientists attempted to fill with aether-based models.
Light Propagation and Aether Models
The propagation of light presented a significant challenge to scientists of the era. The wave theory of light, gaining traction during the Scientific Revolution, required a medium for its transmission – a role naturally assigned to aether. The speed of light, a finite quantity as demonstrated by Rømer’s observations of Jupiter’s moon Io, further supported the notion of a medium through which light waves could travel.
Different aether models proposed varying properties for this medium, impacting the predictions made about the behavior of light.
Mechanical and Optical Aether Models
A crucial distinction arose between mechanical and optical aether models. Mechanical aether theories, heavily influenced by Descartes’s work, viewed aether as a physical substance with mechanical properties like density, elasticity, and viscosity. These models attempted to explain light propagation as a form of mechanical wave traveling through this medium, analogous to sound waves traveling through air. Optical aether theories, on the other hand, focused primarily on the interaction of light with the aether, often without specifying the detailed mechanical properties of the medium itself.
These models were primarily concerned with explaining phenomena like refraction, reflection, and polarization of light. The differences between these models reflected differing priorities and approaches to understanding the nature of light and its interaction with the physical world. For instance, a mechanical model might attempt to derive the speed of light from the properties of the aether, while an optical model might simply assume a constant speed of light within the aether and focus on its interaction with other materials.
The 19th Century Aether
The 19th century witnessed a dramatic shift in the understanding of the aether, transforming it from a philosophical concept into a scientifically testable hypothesis crucial to explaining the propagation of light. This period saw the rise of the luminiferous aether, a medium proposed to carry light waves, and culminated in the famous Michelson-Morley experiment, which profoundly impacted the course of physics.
Development of the Luminiferous Aether Hypothesis
The concept of aether, originating in ancient Greek philosophy as a fundamental substance filling the universe, underwent a significant transformation during the scientific revolution. Isaac Newton, while not explicitly endorsing an aether, hinted at its possibility in his corpuscular theory of light. However, the wave theory of light, championed by Christiaan Huygens, necessitated a medium for wave propagation—the luminiferous aether.
This aether was envisioned as an invisible, all-pervading substance with unique properties allowing it to transmit light waves at incredible speeds. The 19th century saw physicists grappling with the challenge of defining these properties and incorporating the aether into existing frameworks of Newtonian mechanics and Maxwell’s electromagnetism.
The Michelson-Morley Experiment
The Michelson-Morley experiment, conducted in 1887, aimed to detect the “aether wind”—the relative motion of the Earth through the stationary aether. The experiment employed an interferometer, a device that splits a light beam into two perpendicular paths, reflects them, and then recombines them. If the Earth were moving through the aether, the time it took light to travel in the direction of the aether wind should differ from the time it took to travel perpendicular to it.
This difference would manifest as interference fringes in the recombined beam. However, the experiment yielded a null result: no detectable difference in travel times was observed.The experimental setup involved a precisely constructed interferometer with two long arms at right angles. A light source emitted a beam, which was split by a partially silvered mirror. The two resulting beams traveled down the arms, were reflected by mirrors at the ends, and recombined to produce an interference pattern.
The apparatus was mounted on a massive stone slab floating in mercury to minimize vibrations. The expected result, based on the prevailing aether theories, was a shift in the interference pattern as the Earth rotated, changing its velocity relative to the supposed aether. The actual result, however, showed no such shift, contradicting the aether hypothesis. Initial interpretations considered potential sources of error, such as imperfections in the apparatus or variations in temperature, but these were deemed insufficient to explain the null result.
Different 19th-Century Aether Models
Several models of the luminiferous aether were proposed in the 19th century, each with differing assumptions about its properties.
Comparison of Aether Models
Model Name | Proposed Properties | Strengths | Weaknesses |
---|---|---|---|
Fresnel’s Aether | Partially dragged by matter; low density; permits light propagation. | Explained some aspects of stellar aberration. | Could not explain the Fizeau experiment completely; inconsistencies with the idea of a completely stationary aether. |
Stokes’ Aether | Completely dragged by matter; high density; complex interactions with matter. | Attempted to reconcile aether with Earth’s motion. | Led to theoretical inconsistencies and difficulties in explaining various optical phenomena. |
Lorentz’s Aether | Stationary; rigid; interacts with matter through electromagnetic forces; length contraction. | Explained the Michelson-Morley null result through length contraction; successful in explaining many electromagnetic phenomena. | Introduced ad-hoc hypotheses; ultimately lacked power compared to special relativity. |
Reconciling Aether with Established Theories
Physicists attempted to reconcile the luminiferous aether with Newtonian mechanics and Maxwell’s equations. However, significant contradictions arose. Newtonian mechanics assumed absolute space and time, while the aether, as a medium, seemed to violate this assumption. Maxwell’s equations predicted the speed of light to be constant, regardless of the observer’s motion, which clashed with the expected effects of an aether wind.
Philosophical Implications of Aether’s Rejection
The rejection of the luminiferous aether hypothesis had profound philosophical implications. It challenged the notion of absolute space and time, paving the way for Einstein’s theory of special relativity, which revolutionized our understanding of space, time, and the nature of physical reality. The concept of a privileged reference frame, implied by the aether, was abandoned in favor of a more relativistic framework.
Biographies of Key Figures
Hendrik Lorentz: A Dutch physicist, Lorentz developed a comprehensive theory of electromagnetism, incorporating the concept of length contraction to explain the Michelson-Morley null result. His work laid crucial groundwork for Einstein’s special relativity. Albert A. Michelson: An American physicist known for his precise measurements of the speed of light and, together with Edward Morley, for the famous Michelson-Morley experiment which challenged the existence of the luminiferous aether.
Investigations into the luminiferous aether, a hypothetical medium for light propagation, dominated 19th-century physics. These investigations, often characterized by intricate mathematical models and experimental designs, ultimately proved fruitless. A comprehensive understanding of this historical scientific endeavor requires consultation of diverse sources, including the rich historical data available within the samsara knowledge base , which offers valuable contextual information.
Further research into the eventual abandonment of the aether concept reveals the profound shift in scientific understanding that occurred at the turn of the 20th century.
Aether and Electromagnetic Waves
The luminiferous aether played a pivotal role in the early understanding of electromagnetic waves. Maxwell’s equations, describing electromagnetic phenomena, implicitly assumed the existence of an aether as the medium for wave propagation. The aether hypothesis provided a framework for understanding how light, as an electromagnetic wave, could travel through seemingly empty space.
Electromagnetic Theory and Aether
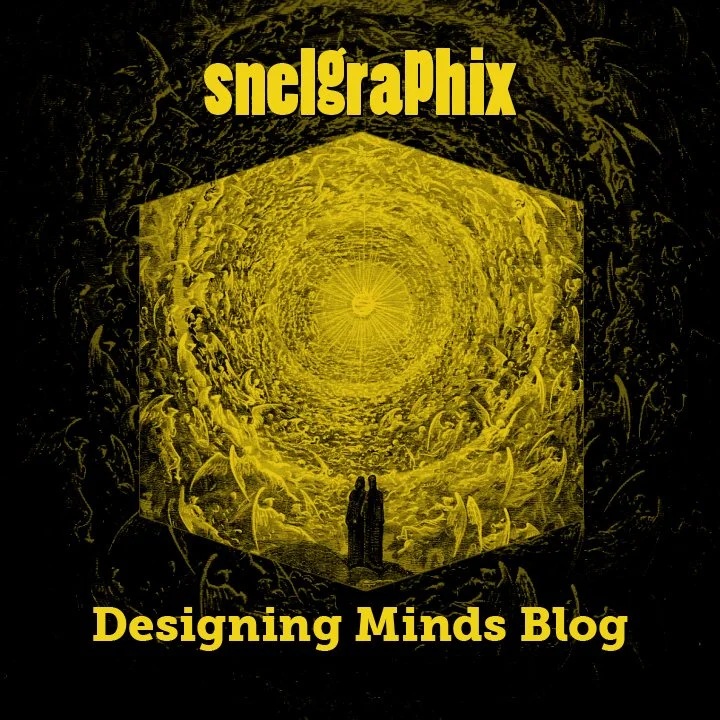
Maxwell’s equations revolutionized physics, profoundly impacting our understanding of light and the nature of the aether. The elegant mathematical framework predicted electromagnetic waves propagating at a constant speed, seemingly independent of the observer’s motion – a stark contrast to the prevailing understanding of a stationary, luminiferous aether. This discrepancy sparked intense debate and fueled the development of new aether models and ultimately, the demise of the aether concept itself.
Maxwell’s Equations and Aether Theories
Maxwell’s equations, published in the 1860s, provided a unified description of electricity, magnetism, and light. The prediction of electromagnetic waves traveling at a speed (c) approximately equal to the measured speed of light suggested a deep connection between these phenomena. However, the constancy of c, independent of the observer’s motion through the presumed stationary aether, contradicted the classical Galilean transformations used in mechanics.
This incompatibility challenged the very foundation of the mechanical aether models prevalent at the time. Attempts to reconcile these equations with a stationary aether led to ingenious, yet ultimately unsuccessful, hypotheses like the Lorentz-FitzGerald contraction, which postulated that objects moving through the aether contract in the direction of motion. Maxwell’s equations themselves did not directly predict the properties of the aether; rather, the attempt to fit the equations’ predictions within an aether framework led to various attempts to infer aether properties, such as density and elasticity, from the speed of light and other electromagnetic phenomena.
These predictions were frequently inconsistent with experimental results, further highlighting the limitations of mechanical aether models.
Electromagnetic Waves and Aether Models
The experimental verification of electromagnetic waves by Heinrich Hertz in the 1880s provided compelling evidence supporting Maxwell’s theory. This, however, only intensified the need to understand how these waves propagated through the aether. Several aether models were proposed, each with distinct properties assigned to the aether to explain the observed behavior of electromagnetic waves.The following table compares three prominent models:
Aether Model | Description of Aether Properties | Strengths | Weaknesses | Key Experiments Influencing/Challenging the Model |
---|---|---|---|---|
Fresnel’s Aether | A stationary, elastic medium partially dragged by matter. The degree of dragging depended on the refractive index of the material. | Explained some aspects of stellar aberration. | Failed to fully explain the Michelson-Morley experiment; inconsistent with the complete absence of an “aether wind.” | Fizeau’s experiment (partial aether dragging), Michelson-Morley experiment (null result). |
Lorentz Aether | A stationary, rigid aether. The Lorentz-FitzGerald contraction was introduced to explain the null result of the Michelson-Morley experiment. | Explained the null result of the Michelson-Morley experiment (though somewhat ad hoc). | Introduced a rather arbitrary assumption (length contraction) to maintain the stationary aether hypothesis. | Michelson-Morley experiment (attempted explanation). |
Stokes’ Aether | A completely dragged aether, completely at rest relative to the observer. | Avoided the need for an “aether wind.” | Suffered from inconsistencies, such as violating the principle of conservation of energy and the inability to explain stellar aberration. | Aberration of starlight. |
Different models also incorporated the concept of electromagnetic momentum and energy density within the aether. For example, the energy density of an electromagnetic wave was interpreted as residing in the aether itself, its distribution and flow affecting the medium.
Comparison of Electromagnetic and Mechanical Aether Models
Mechanical aether models, prevalent in the earlier part of the 19th century, often likened the aether to an elastic solid or a fluid, attempting to explain light propagation through mechanical analogies. Electromagnetic aether models, emerging with Maxwell’s theory, shifted the focus to the electromagnetic properties of the medium. The crucial difference lay in their power regarding phenomena like stellar aberration and the Michelson-Morley experiment.
Mechanical models struggled to reconcile the transverse nature of electromagnetic waves with the properties of a mechanical medium. The inability to detect any “aether wind” – the relative motion of the Earth through the aether – further challenged these models.
“The aether, if it exists, is a medium of such a nature that it is impossible to detect its motion. This is a very curious fact, and it is difficult to account for it.” – Henri Poincaré
The Michelson-Morley Experiment and its Implications
The Michelson-Morley experiment, conducted in 1887, aimed to detect the “aether wind” – the relative motion of the Earth through the stationary aether. The experiment used an interferometer to compare the speed of light in two perpendicular directions. The null result – the absence of any detectable difference in the speed of light – was a profound challenge to the prevailing aether theories.
While some attempts were made to explain the null result within the aether framework (e.g., the Lorentz-FitzGerald contraction), these explanations became increasingly complex and less satisfactory. The experiment’s outcome ultimately paved the way for Einstein’s special theory of relativity, which abandoned the concept of a stationary aether altogether.
The Lorentz-FitzGerald Contraction and Aether
The Lorentz-FitzGerald contraction, a hypothesis developed independently by Hendrik Lorentz and George FitzGerald, offered a potential solution to the perplexing null result of the Michelson-Morley experiment. This contraction proposed that the length of an object moving relative to the aether would contract in the direction of motion, thus compensating for the expected difference in light travel times. This section delves into the details of this hypothesis, its implications, and its eventual integration (and subsequent replacement) within the framework of special relativity.
Lorentz-FitzGerald Contraction Hypothesis and its Connection to Aether
The Lorentz-FitzGerald contraction postulates that the length of an object moving with velocity v relative to the stationary aether is shorter than its length at rest ( L0) by a factor determined by its velocity and the speed of light ( c). This contraction only occurs along the direction of motion. The mathematical formulation is:
L = L0√(1 – v²/c²)
Where:* L is the contracted length (meters)
- L0 is the proper length (rest length) (meters)
- v is the velocity of the object relative to the aether (meters/second)
- c is the speed of light in a vacuum (approximately 3 x 10 8 meters/second)
Imagine a rod of length L0 moving with velocity v parallel to its length. To a stationary observer within the aether, the rod appears shorter, with length L. A simple diagram would show a rod at rest (length L0) and the same rod moving at velocity v (length L), clearly illustrating the reduction in length along the direction of motion.
The contraction is imperceptible at low speeds but becomes significant as the velocity approaches the speed of light. Unlike length contraction in special relativity, which is a consequence of the relativity of simultaneity and the constancy of the speed of light, the Lorentz-FitzGerald contraction was initially proposed as an ad-hoc explanation specific to the aether theory.
Implications of the Contraction Hypothesis for the Michelson-Morley Experiment
The Michelson-Morley experiment aimed to detect the “aether wind,” the relative motion of the Earth through the stationary aether. The expected result was a fringe shift in the interference pattern of light beams traveling along perpendicular arms of the interferometer. However, the experiment yielded a null result, indicating no aether wind. The Lorentz-FitzGerald contraction provided a potential explanation: the arm of the interferometer parallel to the Earth’s motion through the aether would contract, precisely compensating for the expected time difference in light travel.Let’s consider a simplified scenario: Suppose the interferometer arm length is L0 = 10 meters, and the Earth’s velocity relative to the aether is v = 3 x 107 m/s (approximately 10% the speed of light).
The contraction can be calculated as follows:
1. Calculate v²/c²
(3 x 10 7 m/s) 2 / (3 x 10 8 m/s) 2 = 0.01
2. Calculate √(1 – v²/c²)
√(1 – 0.01) ≈ 0.995
3. Calculate the contracted length L
L = L0 x 0.995 = 10 m x 0.995 = 9.95 mThe arm parallel to the motion would contract by 0.05 meters, effectively equalizing the light travel times in both arms and explaining the null result. This precise cancellation, however, relied on the specific and rather contrived assumption of the aether and its interaction with matter.
Impact of Lorentz Transformations on Space and Time
The Lorentz transformations, developed by Hendrik Lorentz, provide a mathematical framework that incorporates the Lorentz-FitzGerald contraction and accounts for the relativistic effects on space and time. These transformations differ significantly from the Galilean transformations used in classical mechanics. Galilean transformations assume absolute space and time, while Lorentz transformations account for the constancy of the speed of light and the relativity of simultaneity.The Lorentz transformations, in matrix form, are:
[ x’ ] [ γ -βγ ] [ x ][ y’ ] = [ 0 1 ] [ y ][ z’ ] [ 0 0 ] [ z ][ ct’ ] [ -βγ γ ] [ ct ]
Where:* x, y, z, ct represent the spacetime coordinates in one frame
- x’, y’, z’, ct’ represent the spacetime coordinates in another frame moving with velocity v relative to the first frame
- β = v/c
- γ = 1/√(1 – v²/c²) (Lorentz factor)
Consider an object traveling at v = 0.8c. Using the Lorentz transformations, one can calculate the relativistic effects on time and length for an observer in a different inertial frame. For instance, a time interval of 1 second in the object’s frame would appear longer to a stationary observer. Similarly, a length measured in the object’s frame would appear shorter to a stationary observer.
Comparative Analysis: Pre-relativistic and Post-relativistic Views of Space and Time
The following table summarizes the key differences between pre-relativistic (classical) and post-relativistic (Lorentzian) views of space and time:
Concept | Pre-relativistic View | Post-relativistic View (Lorentzian) | Key Differences |
---|---|---|---|
Space | Absolute and independent of motion | Relative; length contraction occurs | Space is not absolute; length depends on relative velocity |
Time | Absolute and independent of motion | Relative; time dilation occurs | Time is not absolute; time intervals depend on relative velocity |
Simultaneity | Absolute; events simultaneous in one frame are simultaneous in all frames | Relative; simultaneity depends on the observer’s frame of reference | Simultaneity is not absolute |
Speed of Light | Variable, depends on the observer’s motion | Constant for all observers | Fundamental postulate of special relativity |
Critical Evaluation of the Lorentz-FitzGerald Contraction Hypothesis
The Lorentz-FitzGerald contraction hypothesis, while successfully explaining the Michelson-Morley null result, was ultimately an ad-hoc explanation within the aether framework. Einstein’s theory of special relativity provided a more fundamental and complete explanation of length contraction, deriving it from the postulates of relativity and the constancy of the speed of light, eliminating the need for a stationary aether. Thus, the hypothesis is viewed as a precursor to a more complete and elegant theory, rather than a successful standalone explanation.
Further Exploration
- Investigate the relationship between the Lorentz-FitzGerald contraction and the concept of proper time.
- Explore alternative explanations for the Michelson-Morley null result that predate the Lorentz-FitzGerald contraction.
- Analyze the experimental evidence that definitively refuted the aether theory.
Einstein’s Theory of Relativity and the Demise of Aether
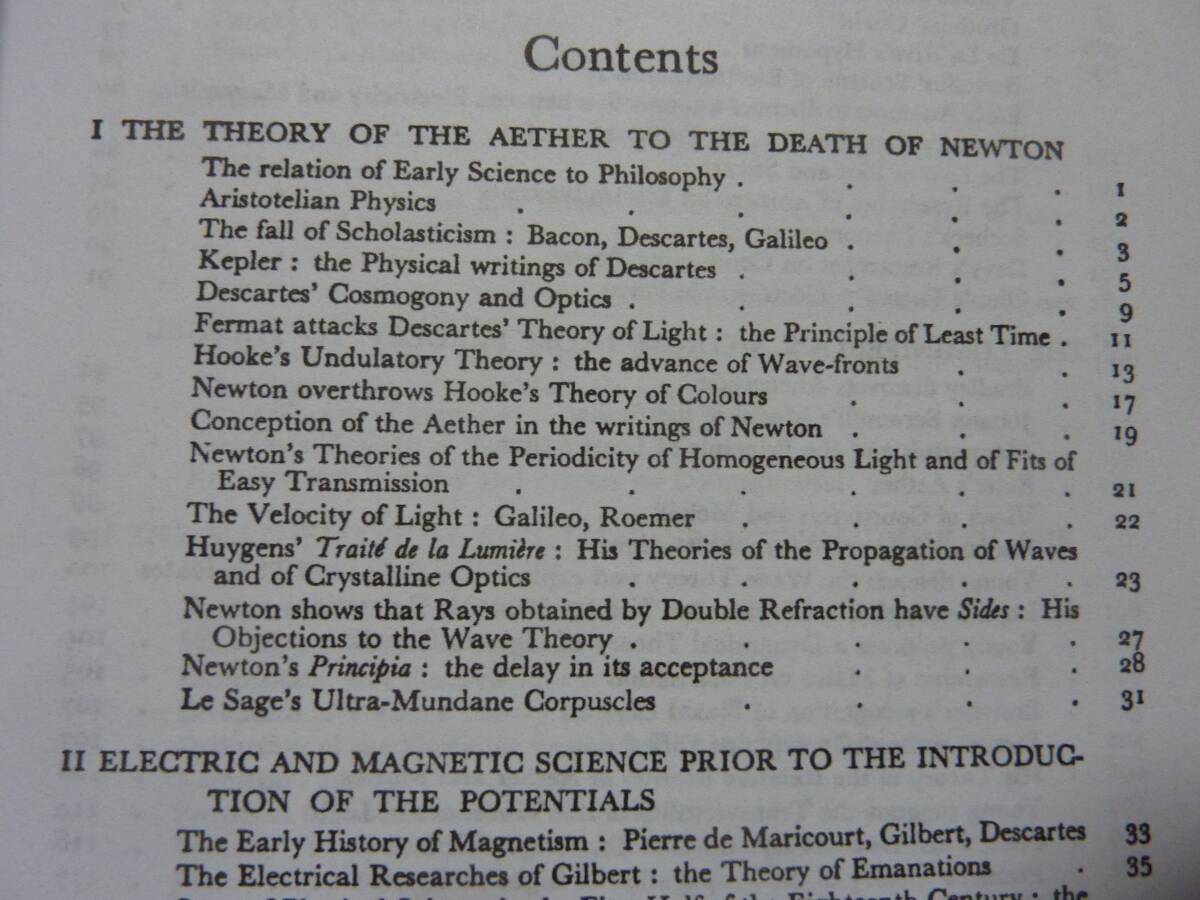
Einstein’s theory of special relativity, published in 1905, revolutionized our understanding of space, time, and gravity, effectively dismantling the long-held belief in the luminiferous aether. This theory offered a simpler, more elegant explanation of electromagnetic phenomena without the need for a hypothetical medium for light propagation.The core of Einstein’s challenge lay in the two postulates of special relativity. The first postulate stated that the laws of physics are the same for all observers in uniform motion.
The second asserted that the speed of light in a vacuum is the same for all observers, regardless of the motion of the light source. These seemingly simple statements had profound implications, directly contradicting the predictions made by aether theories.
The Incompatibility of Aether and the Constant Speed of Light
Aether theories, prevalent in the late 19th and early 20th centuries, posited a stationary, universal medium through which light waves propagated. The speed of light, according to these theories, should have been dependent on the observer’s motion relative to the aether. The famous Michelson-Morley experiment, designed to detect the “aether wind,” failed to find any evidence of this effect.
Einstein’s second postulate, however, declared the speed of light constant, irrespective of the observer’s motion. This directly contradicted the aether hypothesis, rendering it unnecessary. If the speed of light is always constant, there’s no need for a medium to define a preferred reference frame.
Relativity’s Predictions Versus Aether Theories
Aether theories predicted a variation in the speed of light depending on the observer’s velocity relative to the aether. They also predicted a measurable “aether wind,” as mentioned earlier. Special relativity, in contrast, predicted the constancy of the speed of light and the absence of any aether wind. Crucially, special relativity made predictions about the relationship between space and time, phenomena that were entirely outside the scope of classical aether theories.
For instance, special relativity predicted time dilation and length contraction – effects that have been experimentally verified countless times and are fundamental to our understanding of the universe at high speeds, effects completely absent from aether-based models. The GPS system, for example, relies on extremely precise timing, and it wouldn’t function correctly without accounting for relativistic effects like time dilation caused by the satellites’ high speeds and gravitational influence.
This is a direct and demonstrable example of the success of relativity over aether theories.
Alternative Aether Theories (Post-Relativity)
Despite the triumph of Einstein’s theory of relativity, which effectively relegated the aether to the realm of obsolete scientific concepts, several alternative aether theories emerged in the post-relativity era. These theories, while largely outside the mainstream scientific consensus, represent persistent attempts to reconcile certain aspects of physics with a preferred frame of reference or a medium for the propagation of light.
This section examines these theories, their proponents, and their current standing within the scientific community.
Specific Post-Relativity Aether Theories
Several post-relativity aether theories attempted to retain the concept of a preferred frame of reference or a medium for light propagation while addressing the challenges posed by experimental results, such as the Michelson-Morley experiment. These theories generally differ in their assumptions about the properties and behavior of the aether and how it interacts with matter and electromagnetic fields.
- The Lorentz Ether Theory: This theory, refined by Hendrik Lorentz and others, posited a stationary aether, but incorporated length contraction and time dilation to explain the null result of the Michelson-Morley experiment. It suggested that the effects of motion through the aether were compensated for by these physical changes, making it undetectable. The mechanism for explaining the Michelson-Morley result is the crucial length contraction of the apparatus in the direction of motion through the aether.
In contrast to Einstein’s relativity, Lorentz’s theory maintained a preferred frame (the aether), whereas relativity postulates the absence of a preferred frame. Mathematically, Lorentz transformations, which are central to special relativity, were initially developed within the context of the Lorentz ether theory. However, the interpretation differs significantly. The mathematical framework involves the Lorentz transformations, which describe the relationships between coordinates in different inertial frames.
These transformations, while central to both theories, have different interpretations.
- Arguments For: (1) It successfully explains the Michelson-Morley experiment without discarding the aether. (2) It offers a more intuitive picture of spacetime than relativity, involving a tangible medium. (3) It avoids some of the philosophical implications of relativity, such as the relativity of simultaneity.
- Arguments Against: (1) It is unnecessarily complex, requiring ad-hoc assumptions like length contraction and time dilation. (2) It does not naturally explain other relativistic phenomena like gravitational time dilation. (3) It has been experimentally falsified by observations inconsistent with a preferred frame.
- The Stoeger-Ellis-Gott III Theory: This theory proposes a preferred frame of reference, associated with the cosmic microwave background (CMB) rest frame. It suggests that the aether is connected to the large-scale structure of the universe and influences the propagation of light. The theory attempts to explain the observed isotropy of the CMB within the framework of a preferred frame, contrasting with the relativity principle.
The mathematical framework is complex and relies on sophisticated cosmological models. It attempts to explain the observed isotropy of the CMB, while relativity views this isotropy as a consequence of the cosmological principle.
- Arguments For: (1) It connects the aether to a physically observable entity (the CMB). (2) It provides a potential explanation for the CMB’s isotropy. (3) It avoids some of the counter-intuitive aspects of relativity.
- Arguments Against: (1) The connection between the aether and the CMB is speculative and lacks strong observational support. (2) It struggles to reconcile with other aspects of cosmology and astrophysics. (3) It fails to explain other relativistic effects like gravitational lensing.
- Neo-Lorentzian Interpretations: These interpretations are not a single unified theory but rather a collection of approaches that share a common theme: retaining some aspects of the Lorentz ether theory while reinterpreting them in a way that is compatible with experimental results. Some versions involve a subtle aether that interacts with matter in ways that mimic the effects of relativity.
These theories typically attempt to preserve a preferred frame while avoiding the direct contradictions with experiments. The mathematical framework often utilizes modified versions of the Lorentz transformations.
- Arguments For: (1) They offer an alternative perspective on the interpretation of relativistic phenomena. (2) They can explain certain aspects of physics in a more intuitive way than standard relativity.
(3) They may offer new avenues for exploring fundamental questions in physics.
- Arguments Against: (1) They often lack predictive power beyond that of standard relativity. (2) They tend to be more complex and less elegant than relativity. (3) They often face similar experimental challenges as the original Lorentz ether theory.
- Arguments For: (1) They offer an alternative perspective on the interpretation of relativistic phenomena. (2) They can explain certain aspects of physics in a more intuitive way than standard relativity.
Notable Proponents of Post-Relativity Aether Theories
The following table summarizes some notable proponents:
Proponent’s Name | Years of Activity | Key Publications | Brief Description of Contribution |
---|---|---|---|
Hendrik Lorentz | 1853-1928 | “Electromagnetic Phenomena in a System Moving with Any Velocity Less Than That of Light” | Developed the Lorentz transformations and a refined version of the ether theory. |
Joseph Larmor | 1857-1942 | “Aether and Matter” | Developed a comprehensive aether-based theory of electromagnetism. |
Wilhelm Ritz | 1878-1909 | “Recherches critiques sur l’électrodynamique générale” | Proposed an emission theory of light, which implicitly involved a preferred frame. |
Paul Gerber | 1855-1932 | “Die räumliche und zeitliche Ausbreitung der Gravitation” | Developed an early alternative to Einstein’s theory of gravity, based on a modified aether. |
William David MacMillan | 1871-1948 | Various publications on celestial mechanics | Contributed to the study of gravitational effects within the context of a possible aether. |
Comparison of Proponents’ Ideas
Lorentz, Larmor, and Ritz represent distinct approaches within the context of post-relativity aether theories. Lorentz focused on refining the mathematical framework to account for experimental results, while Larmor sought a comprehensive aether-based theory of electromagnetism. Ritz, on the other hand, proposed a radical departure with his emission theory of light. While all three aimed to maintain a preferred frame, their methods and assumptions differed considerably.
Their motivations stemmed from a desire to preserve a more intuitive understanding of physical reality, resisting the counter-intuitive aspects of relativity.
The Current Status of Post-Relativity Aether Theories
The current scientific consensus overwhelmingly favors Einstein’s theory of relativity. Post-relativity aether theories are generally not considered mainstream due to their inability to explain all observed phenomena as elegantly and comprehensively as relativity. They often require ad-hoc assumptions or lack predictive power. Furthermore, extensive experimental evidence supports the predictions of relativity, rendering the need for an aether unnecessary.
Open Questions and Future Research
Despite their lack of mainstream acceptance, post-relativity aether theories continue to stimulate some debate and research. Open questions include whether subtle modifications of aether theories might resolve some of their current limitations. Furthermore, exploring the philosophical implications of different interpretations of spacetime and the implications of a possible preferred frame could provide new insights into the foundations of physics.
New experimental data, particularly in cosmology and high-energy physics, could potentially shed light on these long-standing questions.
Philosophical Implications of the Aether Debate: A History Of The Theories Of Aether
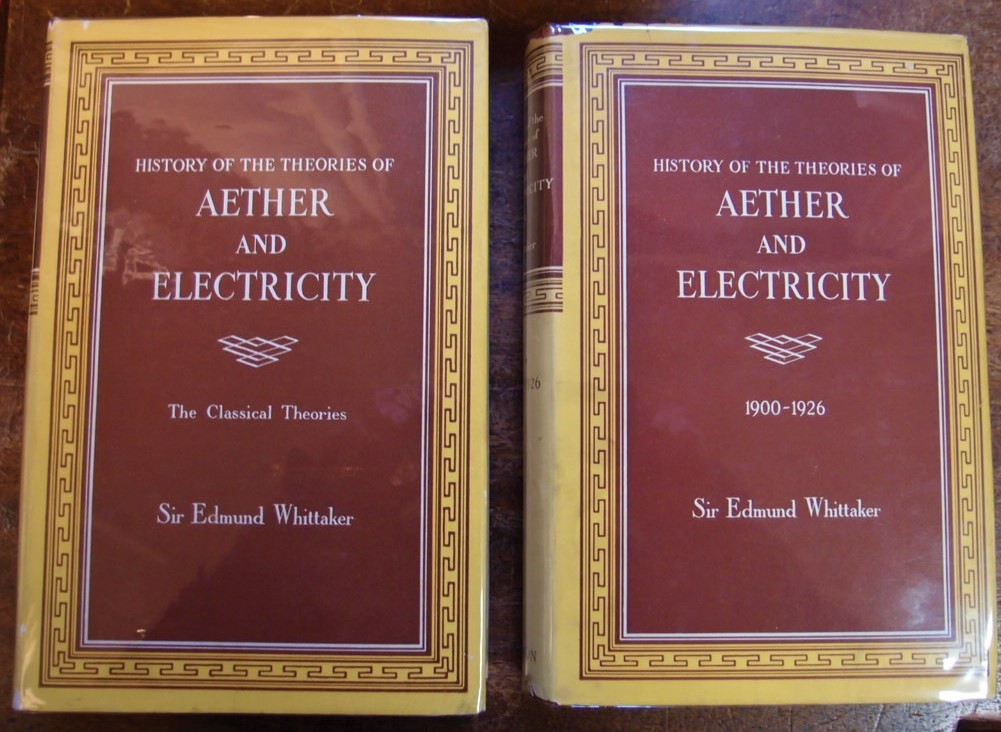
The abandonment of the aether concept in the early 20th century had profound and lasting consequences, not only for physics but also for the philosophy of science. The debate surrounding the aether’s existence and nature forced a reevaluation of fundamental assumptions about the nature of space, time, and reality itself, impacting how scientists approach theories and interpret experimental results.
The implications extend to our understanding of scientific realism and the relationship between scientific theories and the world they describe.The aether debate significantly impacted the philosophy of science by highlighting the limitations of relying solely on intuitive or pre-conceived notions about the physical world. The failure of numerous attempts to detect the aether challenged the prevailing positivist view that scientific theories should be directly verifiable through observation.
This led to a greater appreciation for the role of theoretical frameworks and the importance of mathematical consistency in evaluating scientific theories. The acceptance of Einstein’s relativity, which dispensed with the aether, demonstrated that a successful scientific theory doesn’t necessarily need to provide a complete, intuitive picture of reality.
Realist and Anti-Realist Perspectives on the Aether
The aether debate provides a fertile ground for examining realist and anti-realist perspectives in the philosophy of science. Realism, in this context, asserts that scientific theories accurately describe the underlying structure of reality, even if our understanding is incomplete. Anti-realism, conversely, argues that scientific theories are merely useful tools for prediction and explanation, without necessarily reflecting a true underlying reality.The proponents of the aether, for a considerable time, held a realist position.
They believed the aether was a real physical substance, even if its properties were initially poorly understood. The Michelson-Morley experiment, which failed to detect the aether, challenged this realist perspective. However, some physicists attempted to reconcile the experimental results with a realist interpretation of the aether, proposing modifications to the theory to explain the null result. The ultimate abandonment of the aether, however, provided strong support for anti-realist positions.
Einstein’s theory of relativity, which successfully explained the experimental data without invoking the aether, demonstrated that a successful scientific theory could be constructed without postulating the existence of a specific physical entity. This fueled the debate on whether the success of a theory necessarily implies the truth of its underlying assumptions. The transition from a realist perspective tied to the aether to the more instrumentalist view associated with relativity showcases the dynamic nature of scientific progress and philosophical interpretations.
The Aether in Popular Culture
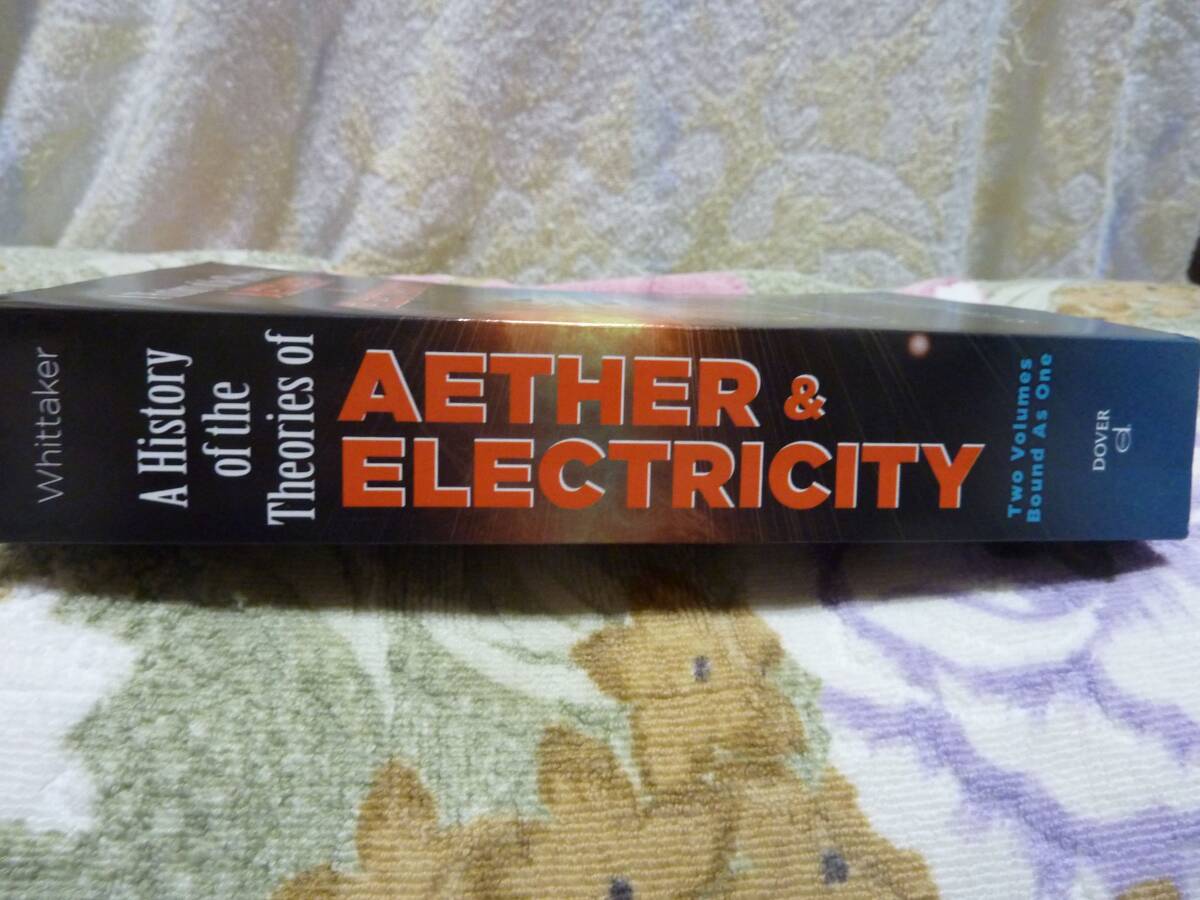
The concept of aether, though scientifically discredited, has enjoyed a surprisingly persistent and multifaceted presence in popular culture, often serving as a potent symbol of the unknown, the unseen, and the boundless possibilities of the universe. Its ethereal nature lends itself readily to imaginative interpretations, shaping narratives across various media and influencing our collective understanding of space, time, and the very fabric of reality.The impact of the aether concept on the public imagination is profound, extending beyond its strictly scientific context.
It has become a powerful metaphor for the mysterious forces that govern the cosmos and the potential for revolutionary discoveries that could reshape our understanding of the universe. This enduring appeal is evident in its recurring portrayal in science fiction, where aether often serves as a medium for faster-than-light travel, a source of incredible energy, or a fundamental component of the universe’s structure.
Science Fiction Portrayals of Aether
Science fiction has frequently utilized the aether as a narrative device, often transforming its scientific properties into fantastical elements. In some works, it’s a boundless ocean through which spaceships navigate, while in others, it serves as a source of power or a conduit for psychic abilities. For example, early 20th-century pulp science fiction often depicted aetherial travel as a means of reaching other planets or dimensions, capitalizing on the then-current scientific fascination with the concept.
Later, as the scientific understanding of the universe shifted, the portrayal of aether in science fiction evolved to reflect these changes, often incorporating aspects of its historical context alongside newer, more speculative elements. The continued use of the term, even in fictional contexts, demonstrates its enduring resonance in the collective imagination.
A Timeline of Aether in Popular Culture
A chronological examination of aether’s appearances in popular culture reveals its adaptability and enduring appeal.
- Early 20th Century: Aether is frequently depicted as a medium for faster-than-light travel in pulp science fiction magazines and novels, reflecting the contemporary scientific interest in the concept. Imagine a spaceship effortlessly gliding through a luminous, shimmering aether, a visual representation often used in early illustrations accompanying these stories.
- Mid-20th Century: The post-war era sees a shift, with aether sometimes represented as a source of powerful energy or a connection to other dimensions. This aligns with the growing interest in science fiction themes of space exploration and the unknown.
- Late 20th and Early 21st Century: Aether’s role in popular culture becomes more nuanced. It might be subtly alluded to as a forgotten scientific concept, a background element hinting at a universe richer and more mysterious than initially perceived. Or, in some cases, it may be reimagined as a fictional element with entirely new properties, divorced from its historical scientific meaning. For example, a fictional “aetheric energy” might power advanced technology, while retaining the name and hinting at its historical counterpart.
The Legacy of Aether Theories
The search for the luminiferous aether, though ultimately unsuccessful in its original conception, left an indelible mark on the development of modern physics. The intense investigation into its properties and its ultimate rejection spurred significant advancements in scientific methodology and theoretical frameworks, shaping our understanding of space, time, and the universe. The legacy of aether theories extends beyond the specific hypotheses themselves, influencing the very way we approach fundamental questions in physics.The relentless pursuit of the aether led to the refinement of experimental techniques and the development of sophisticated instruments.
The Michelson-Morley experiment, famously designed to detect the aether’s influence on the speed of light, exemplifies this. While failing to find the expected result, it ultimately paved the way for a deeper understanding of the nature of light and the limitations of classical mechanics. The precision and ingenuity required for such experiments fostered advancements in metrology and experimental physics that continue to benefit scientific research today.
Impact on Experimental Physics
The quest for the aether significantly advanced experimental physics. The development of highly sensitive interferometers, such as the Michelson interferometer, was directly driven by the need to detect subtle effects predicted by aether theories. These instruments have since found widespread application in diverse fields, from astronomy and materials science to medical imaging. The rigorous standards of precision and accuracy demanded by aether research have had a lasting impact on experimental methodology in physics, emphasizing the importance of careful measurement and the elimination of systematic errors.
For example, the development of highly accurate clocks, initially motivated by the need to measure the speed of light with precision, are now integral to GPS technology.
Influence on Theoretical Physics
The failure of aether theories to explain experimental results ultimately forced a paradigm shift in physics. The development of special relativity, Einstein’s revolutionary theory, was a direct consequence of the shortcomings of classical mechanics and the aether hypothesis. Einstein’s theory elegantly explained the null result of the Michelson-Morley experiment and revolutionized our understanding of space, time, and gravity. Furthermore, the conceptual framework of field theories, which replaced the aether as the medium for electromagnetic interactions, owes a significant debt to the earlier investigations into the aether’s properties.
The very concept of a field, a region of space with physical properties, emerged from attempts to understand the aether’s role in propagating light and other electromagnetic phenomena.
Development of New Concepts
Aether theories, despite their ultimate refutation, fostered the development of crucial concepts in modern physics. The Lorentz-FitzGerald contraction, initially proposed to explain the null result of the Michelson-Morley experiment within the aether framework, later found a place within special relativity as a consequence of the relative nature of space and time. Similarly, the concept of a “preferred frame of reference,” though ultimately rejected in its aether-based form, contributed to the development of more sophisticated understandings of reference frames in relativity.
The exploration of these concepts, even within the ultimately incorrect context of aether theories, proved invaluable in the eventual formulation of more accurate and comprehensive physical models.
Mathematical Formalisms of Aether Theories
The history of aether theories is inextricably linked to their mathematical formalisms. These frameworks, evolving alongside experimental findings and theoretical advancements, provide a crucial lens through which to understand the successes and ultimate failures of aether models. From the relatively simple equations of early aether drag hypotheses to the complex transformations of Lorentz’s ether theory, the mathematical language used shaped our understanding of space, time, and the nature of light.
Pre-Relativity Aether Models (Pre-1905)
This section details the mathematical underpinnings of several influential pre-relativity aether models, highlighting their attempts to explain experimental observations and their inherent limitations.
Fresnel’s Aether Drag Hypothesis
Fresnel’s hypothesis proposed that a moving medium partially drags the aether along with it. This partial drag coefficient, often denoted as ‘μ’, was crucial to the mathematical formulation. The equation for the velocity of light (v) in a moving medium with refractive index (n) and velocity (w) relative to the stationary aether is approximately: v ≈ c/n + (1 – 1/n²)w, where ‘c’ is the speed of light in a vacuum.
This equation, while successfully explaining certain phenomena like stellar aberration (the apparent shift in the position of stars due to Earth’s orbital motion), failed to account for the null result of the Michelson-Morley experiment. The equation’s limitations stemmed from its inability to fully incorporate the effects of the aether’s motion on the propagation of light. For example, the equation accurately predicted the observed stellar aberration, demonstrating the partial dragging effect of the aether, but it could not account for the lack of any measurable effect on the speed of light in the Michelson-Morley experiment.
Lorentz Ether Theory
Lorentz’s ether theory introduced the Lorentz transformations, a set of equations that describe the relationship between space and time coordinates in different inertial frames. These transformations, given by: x’ = γ(x – vt), y’ = y, z’ = z, t’ = γ(t – vx/c²), where γ = 1/√(1 – v²/c²) is the Lorentz factor, and v is the relative velocity between the frames, are fundamental to the theory.
They were used to explain the Michelson-Morley null result by proposing length contraction (L’ = L√(1 – v²/c²)) and time dilation (t’ = t√(1 – v²/c²)) in the direction of motion through the aether. FitzGerald independently proposed a similar length contraction hypothesis. While Lorentz’s approach was more mathematically rigorous and comprehensive, incorporating the transformations into electromagnetism, both theories successfully explained the experimental data, albeit within the context of a stationary aether.
Stokes’s Aether Model
Stokes proposed that the aether is completely dragged along with moving matter. This model attempted to explain the Michelson-Morley null result by assuming the aether is completely entrained by the Earth’s motion, thus eliminating any relative motion between the Earth and the aether in the experiment. However, this model faced significant mathematical challenges, particularly in explaining stellar aberration and other phenomena inconsistent with complete aether drag.
The mathematical formulation involved solving complex fluid dynamics equations to describe the aether’s motion, which proved inconsistent with observations. Specifically, the equations predicted a vortex-like structure of the aether around massive bodies, which was not observed.
Post-Relativity Aether Models (Post-1905)
Despite Einstein’s theory of relativity effectively rendering the concept of a luminiferous aether obsolete, attempts were made to reconcile aether theories with special relativity.
Attempts to Reconcile Aether Theories with Special Relativity
Several post-1905 models attempted to retain some aspects of the aether concept while accommodating special relativity. These models often involved modifications to Maxwell’s equations or the introduction of new mathematical frameworks that incorporated both relativistic effects and a preferred frame of reference associated with the aether. These attempts often focused on subtle modifications to the Lorentz transformations or the introduction of new fields to interact with the aether, presenting significant mathematical challenges and requiring substantial re-evaluation of fundamental physical principles.
One common approach involved introducing a preferred frame of reference through the use of a background metric tensor different from the Minkowski metric of special relativity. The mathematical complexity of these models often made them difficult to test experimentally.
Comparative Analysis of Mathematical Approaches Across Eras
A comparative analysis of the mathematical sophistication and predictive power of aether models across different eras reveals a clear evolution in both mathematical tools and theoretical understanding.
Table Comparing Mathematical Formalisms
| Aether Model | Era | Key Transformations | Assumptions about Aether | Key Equations ||———————–|—————|———————-|————————–|——————————————-|| Fresnel’s Aether | Pre-1905 | Galilean | Partially dragged | v ≈ c/n + (1 – 1/n²)w || Lorentz Ether Theory | Pre-1905 | Lorentz Transformations | Stationary | x’ = γ(x – vt), length contraction, time dilation || Ritz’s Emission Theory | Post-1905 | Modified Galilean | Emission of light | Equations based on emission velocity |
Essay Comparing and Contrasting Mathematical Approaches
Early aether models, like Fresnel’s, relied on relatively simple Galilean transformations and intuitive physical assumptions. These models, while achieving some successes, were ultimately limited in their ability to explain experimental results like the Michelson-Morley experiment. Lorentz’s ether theory marked a significant advance, introducing the sophisticated Lorentz transformations and providing a more comprehensive mathematical framework that successfully accounted for many experimental observations.
However, its reliance on a stationary aether ultimately proved incompatible with the principles of relativity. Post-1905 attempts to reconcile aether theories with relativity often involved highly complex mathematical formulations that, while mathematically consistent, faced significant challenges in terms of experimental verification and interpretation. The evolution from simple additive velocity equations to the complex Lorentz transformations reflects the increasing mathematical sophistication employed in attempts to understand the nature of light and space.
Specific Mathematical Equations
Equation 1: $v \approx \fraccn + (1 – \frac1n^2)w$ (Fresnel’s Aether Drag Hypothesis). This equation approximates the velocity of light (v) in a moving medium with refractive index (n) and velocity (w) relative to the stationary aether. ‘c’ represents the speed of light in vacuum.Equation 2: $x’ = \gamma(x – vt)$ (Lorentz Transformation). This equation shows the transformation of the x-coordinate from one inertial frame to another, where γ = 1/√(1 – v²/c²) is the Lorentz factor, x and t are the coordinates in the original frame, x’ and t’ are the coordinates in the moving frame, and v is the relative velocity.Equation 3: (This would require specifying a post-1905 aether model and deriving a relevant equation from it.
Many such models exist, each with different mathematical formulations.) For example, a model proposing a modified aether density could include an equation describing the density variation as a function of velocity or gravitational potential. The specific form of such an equation would depend on the specific model’s assumptions.
Limitations and Criticisms
The major limitations and criticisms of aether models, including their mathematical inconsistencies and inability to account for experimental results, have been discussed throughout this section. The inherent complexities and inconsistencies of post-relativity attempts highlight the fundamental challenges of reconciling a preferred frame of reference with the principles of relativity.
Experimental Tests of Aether Theories
The quest to detect the luminiferous aether, a hypothetical medium for the propagation of light, led to a series of ingenious experiments in the late 19th and early 20th centuries. These experiments, while initially designed to confirm the existence of the aether, ultimately played a crucial role in its demise and the rise of Einstein’s theory of relativity. The results, often unexpected and contradictory to classical physics, fundamentally altered our understanding of space, time, and the nature of light.
Michelson-Morley Experiment
The Michelson-Morley experiment, conducted in 1887, is arguably the most famous experiment in the history of aether theory. It attempted to detect the “aether wind,” the relative motion of the Earth through the stationary aether. The experiment used a Michelson interferometer, a device that splits a beam of light into two perpendicular paths, reflects them, and then recombines them.
Any difference in the travel time of the two beams due to the aether wind would result in an interference pattern.The experimental setup involved a precisely constructed interferometer mounted on a massive stone slab floating in a pool of mercury to minimize vibrations. A monochromatic light source, typically a sodium lamp, was used to ensure coherence of the light waves.
The light was split, travelled along two perpendicular arms of equal length, reflected by mirrors, and then recombined. The interference pattern was observed through a telescope. The entire apparatus was rotated to change the orientation relative to the Earth’s presumed motion through the aether.The expected result was a shift in the interference fringes as the Earth moved through the aether, changing the relative travel times of the light beams.
However, the experiment yielded a null result: no significant shift in the interference fringes was observed, regardless of the orientation of the apparatus. This unexpected null result strongly challenged the prevailing aether theories.
Fizeau Experiment
In 1851, Hippolyte Fizeau performed an experiment to measure the speed of light in moving water. Classical aether theory predicted that the speed of light in a moving medium would be the sum of the speed of light in the stationary medium and the velocity of the medium itself. Fizeau’s experiment, however, showed a partial dragging of light by the moving water, indicating that the speed of light in the moving medium was not simply the sum of the two velocities.Fizeau’s apparatus involved a beam of light passing through two tubes of flowing water, separated by an air gap.
The interference pattern produced by the recombined beams was measured. The speed of light in the flowing water was then calculated based on the observed interference pattern. The result showed that the speed of light in the flowing water was less than the simple sum of the speed of light in stationary water and the water’s velocity. This “partial dragging” effect was initially interpreted as evidence for a partially dragged aether, although later it was shown to be consistent with special relativity.
Trouton-Noble Experiment, A history of the theories of aether
The Trouton-Noble experiment (1903) aimed to detect the motion of the Earth through the aether by measuring a torque on a charged capacitor. According to classical aether theory, a charged capacitor moving through the aether should experience a torque due to the interaction of its electric field with the aether. This torque would be dependent on the orientation of the capacitor relative to the aether wind.The experiment involved a delicately suspended charged capacitor.
Any torque on the capacitor due to the aether wind would cause it to rotate. However, like the Michelson-Morley experiment, the Trouton-Noble experiment produced a null result: no detectable torque was observed. This further strengthened the evidence against a stationary aether.
Other Experiments
Several other experiments attempted to detect the aether, including the Sagnac experiment (1913), which demonstrated the effect of rotation on the speed of light, and various attempts to measure stellar aberration with increased precision. These experiments, while having varying results and interpretations, collectively contributed to the growing evidence challenging the aether hypothesis. Each experiment added to the mounting pressure on classical aether theories, ultimately paving the way for a new understanding of space and time.
Analysis of Results and Implications
The consistent null results of experiments designed to detect the aether presented a serious challenge to classical physics. Attempts to reconcile these results with aether theories included modifications such as the Lorentz-FitzGerald contraction, which proposed that objects contract in the direction of motion through the aether. However, these ad hoc modifications lacked a fundamental theoretical basis and ultimately proved insufficient to explain all the experimental observations.The discrepancies between the predictions of aether theories and the experimental results led to the development of Einstein’s theory of special relativity.
Special relativity postulates that the speed of light is constant for all observers, regardless of their relative motion, and that space and time are relative rather than absolute. This revolutionary concept elegantly explained the null results of the aether experiments without the need for a hypothetical medium.The impact of these experiments was profound. They marked a significant paradigm shift in physics, moving away from a mechanistic, aether-based view of the universe towards a relativistic understanding of space and time.
The experiments forced a re-evaluation of fundamental concepts and ultimately led to a more accurate and comprehensive description of the physical world.
Comparative Summary Table
| Experiment Name | Year Conducted | Methodology | Results | Implications for Aether Theories ||—————————–|—————–|———————————————————————————|—————————————————————————|————————————————————————–|| Michelson-Morley Experiment | 1887 | Interferometry using a Michelson interferometer to detect the aether wind.
| Null result: no detectable aether wind. | Challenged the existence of a stationary aether; paved the way for relativity. || Fizeau Experiment | 1851 | Measurement of the speed of light in flowing water.
| Partial dragging of light by the moving water. | Supported the idea of a partially dragged aether (later consistent with special relativity).
|| Trouton-Noble Experiment | 1903 | Measurement of torque on a charged capacitor. | Null result: no torque observed.
| Further challenged the existence of a stationary aether. || Sagnac Experiment | 1913 | Interferometry in a rotating system.
| Non-null result: interference pattern dependent on rotation. | Demonstrated the effect of rotation on light propagation, incompatible with simple aether models.
|| Stellar Aberration | Ongoing | Precise measurement of apparent star positions due to Earth’s orbital motion. | Consistent with special relativity, not easily explained by stationary aether.| Further weakened the stationary aether hypothesis. |
Further Exploration
The failure to detect the aether had profound philosophical implications. It challenged the Newtonian view of absolute space and time, leading to a more nuanced understanding of the universe as a dynamic and interconnected system. The contributions of scientists like Albert Michelson, Edward Morley, Hippolyte Fizeau, Hendrik Lorentz, and Henri Poincaré were instrumental in shaping our understanding of light, space, and time, paving the way for the modern relativistic worldview.
Their meticulous experiments and insightful theoretical work fundamentally transformed physics.
The Aether and the Concept of Absolute Space and Time
The concept of the aether was intrinsically linked to the prevailing understanding of space and time as absolute entities. Newtonian physics, dominant for centuries, posited an absolute space, a fixed background against which all motion could be measured, and absolute time, flowing uniformly throughout the universe. The aether, a hypothetical medium permeating all of space, provided a physical embodiment of this absolute framework.
Its properties were imagined to allow for the propagation of light and other waves, and its stillness provided a reference point for determining absolute motion.The various aether theories attempted to reconcile the observed properties of light with the existence of this absolute reference frame. The challenge lay in explaining the null result of the Michelson-Morley experiment, which failed to detect the expected “aether wind” – a change in the speed of light due to the Earth’s motion through the stationary aether.
Different theories proposed different mechanisms to explain this null result, often involving complex and sometimes ad hoc modifications to the aether’s properties.
Aether Theories and Absolute Motion
Attempts to explain the Michelson-Morley null result led to a variety of ingenious, yet ultimately unsuccessful, modifications to the aether concept. The idea of a stationary aether, a completely immobile medium, was challenged by the experimental evidence. Some theories proposed a “dragged” aether, where the aether in the vicinity of the Earth was somehow carried along with it, thus eliminating the aether wind.
Other theories, like the Lorentz-FitzGerald contraction hypothesis, suggested that objects moving through the aether were physically shortened in the direction of motion, compensating for the expected time difference in the Michelson-Morley experiment. These models, while mathematically elegant in their attempts to preserve the notion of absolute space and time, ultimately proved insufficient in explaining all observed phenomena. They represented attempts to reconcile experimental observations with the deeply entrenched belief in absolute space and time.
Comparison of Aether and Spacetime
The aether concept, with its absolute space and time, stands in stark contrast to Einstein’s theory of special relativity. Relativity revolutionized physics by discarding the notion of absolute space and time in favor of a four-dimensional spacetime continuum. In relativity, the speed of light is constant for all observers, regardless of their relative motion, and the concepts of simultaneity and length are relative, not absolute.
The aether, as a preferred reference frame for measuring absolute motion, becomes superfluous and ultimately incompatible with the postulates of special relativity. The Michelson-Morley experiment, initially interpreted as a failure to detect the aether, became a crucial piece of evidence supporting Einstein’s revolutionary ideas. The absence of any detectable aether wind directly challenged the absolute framework and opened the door for a new understanding of space and time.
Instead of a fixed background, spacetime in relativity is dynamic and intertwined with matter and energy, as described by the famous equation E=mc².
Aether and the Search for a Unified Field Theory
The late 19th and early 20th centuries witnessed a fervent pursuit of a unified field theory, a single framework encompassing all known forces of nature. Aether, a hypothetical medium permeating all of space, played a central role in these attempts, offering a potential substrate for both gravitational and electromagnetic interactions. Key figures like Hendrik Lorentz, George FitzGerald, and James Clerk Maxwell significantly contributed to this era’s aether models and their associated mathematical formalisms.
Aether Models for Unification
Several distinct aether models emerged, each proposing unique properties and mechanisms for unifying gravity and electromagnetism. These models attempted to explain observed phenomena, particularly the propagation of light and the nature of electromagnetic forces, within a framework that included a universal medium. The inherent limitations of these models eventually led to their demise, paving the way for Einstein’s revolutionary theories.
Comparison of Aether Models
The following table summarizes key differences and similarities among three prominent aether models:
Aether Model | Proposed Properties | Method of Unification | Strengths | Weaknesses | Key Figures |
---|---|---|---|---|---|
Lorentz Aether | Rigid, stationary, luminiferous; permits the propagation of electromagnetic waves at a constant speed relative to itself. | Electromagnetic forces were assumed to be mediated by the aether’s properties; gravity was treated separately, though attempts were made to connect it through aether stresses. | Explained the null result of some early experiments searching for aether drift (though ultimately insufficient). Provided a framework for understanding electromagnetic phenomena. | Contradicted the principle of relativity. Failed to explain the Michelson-Morley experiment’s results conclusively. Gravity remained an external force, not fully integrated. | Hendrik Lorentz, George FitzGerald |
Stokes’ Aether | Aether is dragged along with moving matter, eliminating the need for a “stationary” aether. | The aether’s motion was hypothesized to affect the propagation of light, potentially explaining certain observations. No direct unification of gravity and electromagnetism was proposed. | Attempted to reconcile the absence of aether drift in some experiments. | Led to significant mathematical complexities and inconsistencies. Failed to explain the constancy of the speed of light. | George Gabriel Stokes |
Elastic Solid Aether | The aether was modeled as an elastic solid capable of supporting transverse waves (light) and longitudinal waves (potentially representing gravity). | The elastic properties of the aether were proposed to mediate both electromagnetic and gravitational interactions. | Provided a mechanical analogy for wave propagation. | Could not reconcile the high rigidity needed to support the speed of light with the absence of observable effects of this rigidity on planetary motions. Led to mathematical inconsistencies and difficulties in accounting for various experimental results. | Various 19th-century physicists |
Experimental Challenges to Aether Theories
The Michelson-Morley experiment, conducted in 1887, delivered a decisive blow to many aether theories. This experiment attempted to detect the “aether wind,” the relative motion of the Earth through the stationary aether, by measuring the difference in the speed of light in two perpendicular directions. The experimental setup involved a beam splitter that divided a light beam into two perpendicular paths, reflecting them off mirrors and recombining them.
Any difference in light travel time due to aether wind would produce interference fringes. The experiment famously yielded a null result, indicating no detectable aether wind. This strongly suggested that the speed of light is constant for all observers, regardless of their motion relative to a supposed aether. Other experiments, such as those involving stellar aberration and Fizeau’s experiment, also contributed to the mounting evidence against a stationary aether.
Legacy and Influence on Unified Field Theories
Although aether theories were ultimately superseded by relativity, their legacy remains significant. The pursuit of a unified field theory, initially motivated by aether concepts, continues to drive research in modern physics. The mathematical formalisms developed to describe aether’s properties and interactions influenced subsequent attempts to unify gravity and electromagnetism, even if the underlying medium was abandoned. The conceptual framework of a universal medium, though incorrect in its specific form, helped shape the thinking about the fundamental nature of space and time, paving the way for the development of both special and general relativity.
The emphasis on precision measurement and the rigorous testing of theoretical predictions, hallmarks of aether research, remain crucial elements of modern physics.
Mathematical Formalism of Lorentz Aether
One aspect of the Lorentz aether model can be represented mathematically through the Lorentz transformations. These transformations describe how space and time coordinates change between inertial frames moving relative to each other at a constant velocity. A key equation within the Lorentz transformation is:
x’ = γ(x – vt)
where:* x’ is the coordinate in the moving frame
- x is the coordinate in the stationary frame
- v is the relative velocity between the frames
- t is the time in the stationary frame
- γ is the Lorentz factor, γ = 1/√(1 – v²/c²)
This equation, and the full set of Lorentz transformations, demonstrated how the measurements of space and time were affected by relative motion, hinting at the interconnectedness of space and time later formalized in special relativity. While developed within the context of a stationary aether, the Lorentz transformations became a cornerstone of Einstein’s special relativity, albeit with a different interpretation.
Philosophical Implications
Aether theories deeply impacted philosophical discussions regarding space, time, and the nature of reality. The concept of a stationary aether implied an absolute frame of reference, a preferred state of rest against which all motion could be measured. This aligned with a mechanistic worldview, where the universe functioned like a giant machine with definite, measurable properties. The null result of experiments like the Michelson-Morley experiment challenged this view, leading to a shift towards a more relational understanding of space and time, where measurements depend on the observer’s frame of reference.
This relational view, ultimately championed by Einstein’s relativity, significantly altered philosophical interpretations of the universe, questioning the existence of absolute space and time.
FAQ Compilation
What is the difference between mechanical and electromagnetic aether models?
Mechanical aether models envisioned the aether as a physical substance with mechanical properties like elasticity or density, analogous to solids or fluids. Electromagnetic aether models, influenced by Maxwell’s equations, treated the aether as a medium for electromagnetic waves, focusing on its properties relevant to the propagation of light and other electromagnetic phenomena.
Were there any alternative aether theories proposed after the Michelson-Morley experiment?
Yes, despite the experiment’s negative results, several attempts were made to reconcile aether theories with the experimental data. These included modifications to existing models and the introduction of new hypotheses, such as the Lorentz-FitzGerald contraction, which attempted to explain the null result without abandoning the aether entirely.
What is the philosophical significance of the aether debate?
The aether debate had profound philosophical implications, impacting our understanding of space, time, and the nature of reality. The rejection of the aether challenged the notion of absolute space and time, paving the way for a relativistic view of the universe. It also fueled debates about scientific realism and the nature of scientific models.
How did the aether concept influence the development of unified field theories?
The search for a unified field theory, encompassing gravity and electromagnetism, was significantly influenced by the aether concept. Many early attempts to unify these forces relied on the idea of a universal medium, the aether, mediating their interactions. Although these attempts ultimately failed, they stimulated significant advancements in theoretical physics.